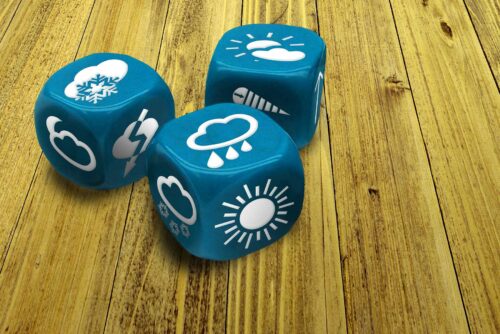
Reimagining Utility Climate Risk Planning
How Regulators and Utilities Can Leverage and Improve Climate Risk Assessment Frameworks to Drive an Affordable and Equitable Transition.
Executive Summary
Today’s grid infrastructure is underprepared for the changing trends in weather- and climate-related disasters. Between 2000-2023, extreme weather accounted for most major power outages in nearly every state. Likewise, utilities are not aligned with a transition to a net zero-carbon economy. Together, such physical climate risks (i.e., risks related to the physical impacts of climate change) and transition climate risks (i.e., risks related to the transition to a zero-carbon economy) threaten the affordability and reliability of clean electricity – the keystone of this future economy.
This insight brief explains how to integrate emerging climate risk assessment frameworks with utility planning processes to enable utilities to navigate a path toward a future grid supporting strong and resilient communities that can weather climate risks (Chapter 2). This process consists of four steps:
Step 1: Identify a list of climate risks and how each might impact the utility business.
Step 2: Assess the impact of climate risks on utility operation quantitatively.
Step 3: Mitigate both exposure to and impacts of climate risks on utility business.
Step 4: Distribute the risks across different stakeholders, including ratepayers and utility investors.
ES Exhibit 1. Utility Climate Risk Planning Process

Focusing on the first two steps, identification and assessment, this insight brief provides an overview of key physical (e.g., extreme heat and cold, drought, flooding, wildfire, hurricane, and rising average temperatures) and transition climate risks (e.g., carbon taxes, CCS retrofits, federal policy, falling technology costs, and emerging technologies) (Chapter 3), and lays out improvements to a climate risk assessment framework detailing how utilities can incorporate robust risk assessment into the planning processes (Chapter 4), with three main components:
- Covers the full range of physical and transition climate risks. It is critical to ensure utilities assess all the risks relevant in a utility service area rather than selecting only the ones that are in favor of their preferred portfolio.
- Defines the methodology for quantifying each risk starting at the asset level and aggregating up to the portfolio level. Specifically, two key datasets — an hourly multi-year utility-specific weather dataset to capture impacts on the system on operational timelines and a 30+ year utility-specific climate dataset to help plan for the chronic realities and extreme events of the future — are critical to ensuring a robust assessment of risks and interannual variability in the modeling efforts.
- Specifies exactly how and where each quantified risk feeds into utility planning models.
Regulators (and other stakeholders working closely with regulators in utility proceedings) are encouraged to consider a suite of actions to ensure utilities take the necessary steps to incorporate climate risks into planning and drive conversations around the trade-offs of risk mitigation versus acceptance in the transition:
- Add climate risk identification and assessment requirements to planning processes.
- Create or leverage existing financial and regulatory incentives for utilities to transition away from fossil assets that present significantly increasing climate risks.
- Work with legislators and financial industry stakeholders to adequately and equitably distribute climate risks among taxpayers, shareholders, and ratepayers, particularly, explore ratepayer- or shareholder-backed self-insurance funds.
As the energy transition accelerates, and extreme weather-related spending along with it, there’s an imperative for regulators and other stakeholders to work with utilities to align on a streamlined and proactive process of identifying, assessing, reducing and distributing climate risks, which create opportunities for a reliable and equitable future.
Chapter 1. Introduction
Utility spending in response to weather- and climate-related forces continues to increase. Extreme weather events including hurricanes, wildfires, extended heat domes, and winter storms as well as shifting climate patterns, such as precipitation, put strain on our aging grid infrastructure. Simultaneously, global market trends further increase supply uncertainty and exacerbate cost volatility in the utility business. Evolving regional regulations introduce opportunities for more effective climate mitigation while also creating challenges for maintaining stability and predictability in long-term planning. Collectively, these factors can increase the cost of running the electric grid, which trickles down to customers’ electricity bills, creating affordability challenges and disproportionately affecting those who live in disadvantaged communities. In California, three major investor-owned utilities have seen wildfire-related costs grow from 2% of the revenue requirement in 2019 to 16% in 2022, and PG&E recently experienced an even more drastic increase in wildfire-related costs — 117% between January 2023 and April 2024, contributing to 24% of the total system revenue requirement as of April 2024.
Effective utility planning can provide the opportunity to manage climate risks if utilities make investment decisions that are informed by a comprehensive risk assessment, but utilities and their regulators must see eye-to-eye to move successfully from risk assessment to risk mitigation. Not every climate resilience measure provides a net cost benefit, which highlights the importance of regulatory prudence reviews. This insight brief presents an overview of climate risks, examines the shortcomings of current utility planning processes, and lays out solutions to improve the analytical framework for assessing climate risks. Implemented successfully, these updates can facilitate climate risk mitigation while achieving reliable and equitable decarbonization of the electric power system.
Chapter 2. Recommended Steps for Utility Climate Risk Planning
Existing utility risk assessment and mitigation processes could accommodate the addition of climate risks. The US Department of Energy and the Electric Power Research Institute (EPRI)’s Climate Resilience and Adaptation Initiative (Climate READi) lay out best practices for vulnerability assessment and resilience planning and for representing climate impacts in power system models, respectively. Exhibit 1 provides a simplified view of typical steps that show up in these and other emerging utility climate risk assessment and planning frameworks. However, we explicitly separate a step focused on distributing climate risk and overlay implicated regulatory venues, which can help findings from assessments impact utility business decisions. Finally, risk assessment processes possess a cyclical nature, with modeling assumptions, progress, and new information continuously informing the next iteration of the process.
Exhibit 1. Utility Climate Risk Planning Process

Briefly, integrating climate risk into integrated resource plans (IRPs), distribution system plans (DSPs), rate cases, and other utility planning processes comprises four main steps:
Step 1: Identify the scope of the analysis (i.e., what planning processes and modeling exercises are implicated), develop a comprehensive set of regionally relevant climate risks, collect necessary data, and determine how each risk might impact the utility business. This can occur during the resource and grid infrastructure planning processes (IRPs, DSPs, risk-specific plans such as wildfire mitigation plans) as well as inform operational decisions for existing assets.
Step 2: Assess the likelihood and severity of impacts stemming from climate risk on the utility business and customers quantitatively. This can occur during the same processes as in Step 1.
Step 3: Mitigate both exposure to and possible impacts associated with climate risks on the utility business using a portfolio of climate resilience methods. We summarize risk reduction actions in two main groups:
- Avoid exposure to climate risks where practical. For example, switching to renewable portfolios avoids exposure to fuel cost volatility.
- Reduce the impacts of climate risks where they cannot be avoided or where avoidance is cost prohibitive. For example, weatherizing power plants reduces the likelihood of frozen power plant components during winter storms.
Risk mitigation actions may occur in a variety of utility planning processes. Resource and grid infrastructure planning processes (IRPs, DSPs, risk-specific plans such as wildfire mitigation plans) offer the most direct venue for implementing climate risk mitigation measures such as generation portfolio shifts and grid hardening. Rate cases, performance-based regulation (PBR) dockets, and fuel recovery dockets also offer opportunities for risk mitigation by creating incentives for utilities to transition away from fossil fuel assets and invest in grid resilience improvement.
Step 4: Distribute the risks across different stakeholders, including ratepayers, utility investors, etc. There are two strategies for risk distribution:
- Reactive measures: After risks materialize and incur costs (e.g., fuel volatility or wildfires increase expenses), stakeholders can explore risk distribution approaches by proposing mechanisms for equitable cost allocation, particularly considering ones that alleviate the energy burden for low- and middle-income (LMI) customers and thereby preventing climate risks from producing a significant affordability crisis in disadvantaged communities. This may occur during rate cases, PBR dockets, fuel cost recovery dockets, etc.
- Proactive measures: Before the risks materialize, stakeholders can alter resource planning rules and incentive structures (e.g., fuel cost sharing) to prevent risks from materializing, balance risks among ratepayers, utilities, and investors, or transfer risks to third parties (e.g., insurance companies). This may occur in traditional resource and grid planning processes (IRPs, DSPs, risk-specific plans such as wildfire mitigation plans) or within the same regulatory venues as the reactive process (i.e., rate cases, PBR dockets, etc.).
While existing climate risk mitigation frameworks imply some distribution of risk within the risk mitigation step, we separate it here to highlight regulators’ central role in this step and point out the additional regulatory venues where risk mitigation action can take place.
This insight brief primarily focuses on Steps 1 and 2 detailing how to identify and assess climate risks. Steps 3 and 4 are discussed briefly in the later sections, with further research needs flagged for future projects.
Chapter 3. Identifying Climate Risks
Climate change poses financial risks to the utility business. Climate related risks fall broadly into two categories: physical risks and transition risks. The Task Force on Climate-related Financial Disclosures (TCFD) provides industry standard recommendations for disclosing climate risks falling into both categories, which have been widely adopted across companies, including some US electric utilities. The US Securities and Exchange Commission (SEC) integrated ideas from the TCFD and the GHG Protocol into their proposed climate risk disclosure requirement, which may become mandatory for public companies in the United States. Given repeated exposure to climate risks and their obligation to serve customers, disclosure is just a starting point for the utility industry.
Physical Climate Risks
Physical climate risks refer to those associated with physical impacts from climate change. Such risks may manifest as acute extreme weather events (such as historic floods, heat waves, and winter storms), or as chronic conditions that represent a new normal (such as rising average temperatures, rising sea levels, and shifting precipitation patterns). These risks can lead to more frequent reduction or disruption in the power supply, impacting reliability and resilience. Further complicating matters, some of these risks are correlated – resulting in events occurring simultaneously or in close succession that result in greater impacts.
Physical climate risks affect all customers, but the impacts of these risks differ among customers. For example, more than 25 million Americans in the Justice40 communities —which are defined as “disadvantaged communities that are marginalized by underinvestment and overburdened by pollution” – are expected to face at least 48 health-threatening heat days per year by 2050. The customers in these underserved communities, who sometimes experience affordability problems during normal days, can face increasingly difficult decisions during extreme weather events as they are driven to restrict their energy use (e.g., limit use of air conditioning during extreme heat), out of fear of unaffordable utility bills. Table A below provides an illustrative sample of the physical climate risks and possible impacts.
Table A. Descriptions of Physical Climate Risks
Transition Climate Risks
Transition climate risks are related to the impending transition to a lower carbon economy, or put differently, risks that laggards will assume by waiting or refusing to take action on the energy transition (e.g., utilities building new gas infrastructure without a plan to switch fuels or including CCS costs in planning models). Transition risks can be broken down into different categories: policy, legal, technological, market, and reputational, all of which are associated with the directive to reduce greenhouse gas emissions and transition to clean energy.
Federal policies such as the Inflation Reduction Act and Infrastructure Investment and Jobs Act have created incentives for zero-carbon technologies. On the other hand, load growth driven by datacenters and advanced manufacturing facilities top the list of drivers behind utilities’ proposals to build new gas plants, creating challenges to meet decarbonization targets set by the utility companies and state legislation. In the meantime, new or revised environmental regulations — such as the Environmental Protection Agency’s (EPA) power plant emission rules — have potentially significant impact on a utility’s operational costs. All these risks contribute to significant uncertainty in the financial impact of utility planning and investment pathways, and if not managed well, these risks may translate into unexpected bill increases. Table B below provides an illustrative sample of the transition climate risks and possible impacts.
These tables are not designed to act as an exhaustive list of all the risks that can possibly impact certain jurisdictions, and each state/utility is expected to conduct a customized assessment to identify the risks that are applicable to the local context. However, it can be a starting point for the regulators and advocates to request utilities to provide evidence around how certain risks may or may not impact their jurisdictions.
Table B. Descriptions of Transition Climate Risks
Chapter 4. Current Practices and Challenges in Climate Risk Assessments
Utilities are starting to explore practices that assess the exposure to climate risks in collaboration with industry experts. EPRI has started to consolidate resources under the Climate READi framework, and independent consultants (e.g., ICF) have started to provide climate risk assessment services to major utilities. Furthermore, some utilities are beginning to assess a subset of climate risks in IRPs, DSPs, or in dedicated risk-specific plans (e.g., wildfire mitigation plans). New York’s ConEd, Southern California Edison, Chicago’s ComEd, and Seattle City Light are leading the way with their climate plans.
Seattle City Light Climate Change Vulnerability Assessment and Adaptation Plan
In 2013, Seattle City Light’s strategic plan established objectives to research the impacts of climate change on the utility and develop an adaptation plan centered around specific mitigation actions. The plan maps eight physical climate hazards to possible impacts to five aspects of utility infrastructure resulting in thirteen total impact pathways. Finally, the report summarized adaptation actions for each aspect of utility infrastructure. While not mandated by any legislative or regulatory body, this plan benefitted from its forward thinking and the detailed description of potential actions. However, it lacks mechanisms for monitoring and enforcement.
While these efforts lay a solid foundation for establishing best practices in climate risk assessment within the planning process, utilities still face challenges in integration and data accessibility, impeding a seamless and comprehensive approach to climate-informed decision-making. For example, separate analyses are usually conducted for each utility modeling exercise (e.g., wind and solar resource forecasts, distributed energy plans, load forecasts, natural gas pipeline expansion and maintenance schedules, etc.) , and the audience and time horizon often differ among these analyses. Furthermore, access to regionally relevant high-resolution climate data and expertise to use this data correctly remains a challenge. This stems from the fact that historical weather data fails to adequately represent future climate risks and access to climate data remains uneven among utilities. Multiple organizations are actively working on solutions to address this data asymmetry – EPRI’s Climate READi and Argonne National Laboratory’s Climate Risk and Resilience (ClimRR) data portal.
Some utilities have multiple planning proceedings, with one or more dedicated to climate risk assessment, often referred to as climate resilience plans, grid security plans, etc. While this represents a solid first step, a siloed process addressing the impacts of climate change introduces additional overhead potentially increasing the difficulty of implementing findings in existing planning and operational processes.
Duke’s Carbon Plan in North Carolina
In September 2023, Duke Energy Carolinas and Duke Energy Progress released a report that provides an assessment of the climate resilience impact of its grid infrastructure investments performed by ICF. However, it focuses only on the physical risk impact of climate change (e.g., high temperature and extreme heat, extreme cold and ice, etc.) without covering transition risks, and it remains unclear what mechanism will encourage Duke to implement any of the recommendations from the analysis. Separately, Duke has been pursuing its resource planning effort through the Carbon Plan proceedings, and although their assessment of forthcoming EPA rules indicated substantial cost impacts, Duke failed to include a discussion around the impact of alternative portfolios.
Without strong regulatory guidance ahead of planning processes, utilities do not have a concrete baseline to move towards risk mitigation and allocation. When a reactive approach is taken after these risks materialize, they translate into costs for ratepayers, resulting in rising bills and stifling energy burdens for LMI customers. In the past few months, PacifiCorp filed multiple requests in several of its jurisdictions to seek deferral of the wildfire-related insurance costs and recover significant insurance premiums. These costs contribute to a portion of the request for a 26.8% rate hike for PacifiCorp’s customers in Idaho and 28.3% increase for customers in Utah, which will further elevate the energy burden in these states. Proactive measures can offer a win-win where they provide a net cost benefit or shift risk away from LMI customers.
Chapter 5. Improving Climate Risk Assessments
This section introduces additional factors that can be deployed by utilities to help better quantify climate risks and assess the distribution of climate risks across different ratepayer classes. Regulators and advocates are likely not equipped with the data and modeling resources to conduct their own analysis that deploys this framework, but they are well positioned to play a critical role in nudging the utilities to deploy or improve climate risk assessments by requesting the utilities to provide information on their analytical approaches and modeling processes. The requirements laid out by the California Public Utilities Commission can offer a starting place for other states considering climate risk assessments. The section “Questions for Regulators and Advocates on Climate Risk Planning” includes a list of targeted questions for regulators and advocates to pose in regulatory proceedings.
A climate risk assessment framework includes the following components:
- Covers the full range of physical and transition climate risks. It is critical to ensure utilities assess all the risks relevant in a utility service area rather than selecting only the ones that are in favor of their preferred portfolio. Physical climate risk identification and assessment requires expert knowledge of weather and climate science as well as how climate events can impact utility assets, whereas transition climate risk assessment requires expert knowledge of policy, economics, and finance. Weather and climate science is new territory for most utilities, so they should engage these new experts in their planning processes.
- Defines the methodology for quantifying each risk starting at the asset level and aggregating up to the portfolio level. When viewed in isolation or for an individual asset, some risks may appear manageable, but if multiple risks materialize at the same time, in quick succession, or cover a large enough part of the portfolio, the picture may change. Core to these methodologies lie two datasets:
- An hourly multi-year utility-specific weather dataset that captures a reasonable distribution of future conditions, which affect the system on operational timelines. Ideally, this dataset combines high-quality historical observational data products and high-resolution downscaled climate data. This dataset acts as the foundation for all load forecasting and production cost modeling runs.
- A 30+ year utility-specific climate dataset that captures the distributions of future temperature, precipitation, wind, and humidity to help plan for the chronic realities and extreme events of the future. This dataset acts as the foundation for long-term planning model runs, and the long-time horizon is required to capture variability across scales (e.g., extreme events, year-to-year variability, El Niño Southern Oscillation (ENSO) intensity, drought patterns, etc.). ENSO provides a key example for the necessity of a long-time horizon in climate datasets: it cycles irregularly every 2-7 years, and its intensity changes on a 10-15 year timeline. Therefore, a dataset spanning less than 7 years may only capture one aspect of the ENSO cycle and any dataset shorter than 15 years may only capture a single level of intensity. Furthermore, a 30-year dataset is hardly adequate for capturing extreme events — containing at best a 1-in-30-year event. While this meets the 1-in-10-year criteria applied in many resource adequacy studies, it fails to capture the 100-year events experienced by so many utilities in the past several years. In other words, extreme event modeling in particular requires a dataset much longer than those traditionally used in utility planning, but is necessary to adequately characterize the range of conditions that proposed 20–50 year assets will experience between today and 2075.
- Specifies exactly how and where each quantified risk feeds into utility planning models. For example, load forecasting, production cost modeling, and capacity expansion modeling for IRPs, DSPs, etc. It can also define a strategy for how the decision-making process addressing uncertainty.
Exhibit 2 below provides a conceptual illustration of how an improved climate risk assessment might affect portfolio selection in an IRP. In the scenario where no climate risk is considered (the “Base Scenario Cost” bar), capacity expansion modeling may produce a fossil-heavy portfolio that’s financially competitive compared to a clean energy portfolio. By integrating a climate risk assessment with capacity expansion and production cost modeling, planners can estimate previously externalized costs (the “Climate Risk Impact” bar), which may result in a lower overall cost for the clean energy portfolio.
Exhibit 2. Illustrative Risk Assessment Comparison
A large fraction of financial risks come from market uncertainties and fluctuating operating expenses, which are costs that are mostly classified as energy-related (i.e., variable) costs. Energy-related costs have a disproportionate impact on residential customers because, most often, the largest share of energy-related costs is allocated to the residential class in the cost allocation process. Additionally, commercial and industrial customers, who pay a larger fraction of demand-related costs through demand charges, have accessibility to hedging and direct procurement opportunities, which insulate them from the impact of high energy-related costs. In other words, clean energy portfolios – much less prone to market fluctuation due to fuel cost uncertainties and overall market uncertainties once they are in service – create more robust benefits for residential customers, including the ones that live in disadvantaged communities.
Quantitative Example of Deploying an Improved Modeling Framework
To demonstrate how this improved modeling framework works, we present an example that captures a subset of diverse climate risks and how their impacts are layered upon one another, creating a more expensive future compared to a simple baseline cost assumption. In this example, we create two sample portfolios: The gas scenario builds new gas resources, and the renewable scenario builds equal parts wind and solar with the same capacity as the gas resources. We assume that the rest of the portfolio is identical between the two scenarios, and therefore exclude it for brevity. Exhibit 3 below illustrates the ratepayer cost savings in the renewable scenario relative to the gas scenario, when both scenarios are exposed to a set of climate risks.Exhibit 3. Total Climate Risk Assessment Cost Comparison
Digging into the possible impact of each risk, CCS retrofits, carbon taxes, and natural gas prices stand out as the largest drivers of cost increase in the gas scenario, highlighting the uncertainty surrounding tightening carbon regulations and EPA rules. Carbon taxes or regulations are often qualitatively discussed or quantitatively assessed in sensitivity scenarios that do not directly inform the resource portfolio decision. Volatile natural gas prices and purchase cost increases, on the other hand, are often reflected in the IRP modeling, but utilities systematically underestimate the impact of extreme weather events on gas prices and purchase costs, leading to an insufficient picture of these risks in preferred portfolio resource modeling. We used moderate assumptions in this example, yet the gas scenario costs exceed the renewables scenario costs by 68%. In a complete implementation, each risk would ideally be represented by a probabilistic distribution. Methodological details underlying this example are provided in Appendix 1 and more detailed results are provided in Appendix 2.
Questions for Regulators and Advocates on Climate Risk Planning
The questions that we lay out in this section represent a starting point to help regulators investigate a utility’s process for identifying and assessing climate risks but are by no means exhaustive.
Table C. Discovery Questions for Regulators and Advocates
PUC Review Expectation
Discovery Questions
High-level Consideration: How is the utility accounting for physical climate risks during planning?
Utility employs an hourly multi-year utility-specific weather dataset.
- Please describe the historical weather data used in all modeling activities including the spatial and temporal resolution, the geographic coverage, and the time horizon.
- If the dataset’s temporal resolution is lower than hourly, explain what steps you’ve taken to capture the relevant intraday variability in all meteorological variables.
- If the dataset covers only a single year, explain what steps you’ve taken to account for the full range of past extreme events.
- If the temporal resolution is greater than 2km, explain what steps you’ve taken to correct for terrain effects lost at lower resolutions.
Utility employs a 30+ year utility-specific climate dataset.
- Please describe your process for downscaling climate data to produce a relevant dataset for your jurisdiction (i.e., what climate data product(s) are you using, is your process statistical or dynamical, what are the final spatial and temporal resolution, the geographic coverage, and the time horizon of the resulting dataset).
- Describe what combination of historical and projected data you use in load forecasting, capacity expansion, and projection cost modeling activities. If you are not using any projected data, explain how you’re capturing future conditions. If you are using projected data, explain the process by which you projected that data and the relationship between this data and your downscaled climate data.
Utility performs an assessment of all relevant physical climate risks.
- Provide statistics covering the frequency and severity of all relevant extreme weather events (e.g., heat waves, winter storms, flooding, hurricanes, or wildfires) over the entire horizon of your planning processes. If both the frequency and severity are not increasing over long time horizons, justify this trend using evidence from your climate dataset.
Utility load forecast is built upon the weather and climate datasets.
- Describe exactly how your weather and climate datasets impact your load forecast. Does your load forecast account for changing technology adoption trends (e.g., air conditioning, heat pumps, air purifiers, etc.) stemming from a gradually warming world? If not, show why using evidence from your climate dataset.
High-level Consideration: How is the utility accounting for transition climate risks during planning?
Utility performs an assessment of all relevant transition climate risks.
- Discuss individually which policy and regulatory, legal, technological, operational, and market risks you’re tracking. If you’re not tracking any risks in a certain category provide a justification.
Utility performs an assessment of all relevant policy and regulatory risks.
- Provide a detailed list of individual policies – at both the state and federal levels – that you’ve included in long-term planning.
- Did you model any type of carbon price? If so, provide explanations for the range of prices used in modeling. If you only included a carbon price in some scenarios, explain your reasoning.
Utility financial forecast includes spending on climate- and weather-related litigation.
- Provide a full list of past climate- and weather-related litigation claims, and report how much litigation-related spending appears in your forecast.
- If your forecast doesn’t show increased spending on climate- and weather-related litigation on a forward-looking basis, explain what mitigation efforts you’ve completed or planned to reduce such liabilities and their associated costs.
Utility performs technology cost sensitivity analyses.
- Explain your methodology for forecasting capital and operational expenditures for new units particularly the origin of your assumptions around clean technologies with rapidly decreasing prices (e.g., storage, solar, or wind) and emerging clean firm technologies with highly uncertain prices (e.g., long-duration storage, small modular reactors, or advanced geothermal).
Utility performs an assessment of the transition strategy of new or existing fossil resources.
- If an IRP includes continued operation of existing fossil assets or proposed new gas, provide a detailed description of your plan to comply with future rules limiting carbon dioxide emissions for both new and existing plants.
- If the plan involves fuel-switching to hydrogen, include plans and costs associated with delivering hydrogen to the proposed natural gas facility including the proposed source of the hydrogen.
- If the plan involves CCS, include plans and costs associated with transporting and sequestering CO2 produced by the proposed natural gas facility. These transport costs should differ based on the distance to the long-term CO2 sequestration facility.
- If the plan involves CCS, describe in detail locations and capacities of long-term CO2 sequestration facilities. If you plan to use a third-party off taker, report their current offtake capacity as a percentage of the amount you expect them to offtake from your power plants.
Utility performs an assessment of all relevant market risks.
- Explain quantitatively how you forecast natural gas prices over the planning horizon.
- If the price forecast is single-valued (i.e., not probabilistic), provide a justification for neglecting the uncertainty inherent in the price forecast.
- Would a persistently higher natural gas price result in different resource builds or accelerated retirements compared to your current/preferred portfolio?
- Explain qualitatively and quantitatively how you account for natural gas supply disruptions in your planning models.
- Explain how costs incurred during short-run (i.e., weather-driven) supply disruptions and long-run (i.e., geopolitics-driven) supply disruptions would impact cash flows and ratepayer bills.
- Explain quantitatively how you forecast wholesale electricity prices over the planning horizon.
- If the price forecast is single-valued (i.e., not probabilistic), provide a justification for neglecting the uncertainty inherent in the price forecast.
- Would persistently higher wholesale electricity prices result in different resource builds compared to your current/preferred portfolio?
Chapter 6. What’s Next: What Regulators Can Do to Address Climate Risks
The improvements discussed above can help utilities gain a better understanding of what climate risks exist, how they manifest in operations, and how to quantify potential impacts during the planning process. However, in no jurisdiction has a comprehensive framework, as outlined in this paper, been implemented. Fortunately, regulators and policymakers may have solutions at their disposal to address any potential gaps:
1. Require climate risk identification and assessment requirements to planning processes.
One of the best opportunities for a utility to identify climate risks and take steps to avoid or reduce those risks are forward-looking planning proceedings, including resource and distribution planning. Where these processes are already mandated, they typically require utilities to conduct load forecasting, commodity price forecasting, and are a platform by which the utility makes decision on resources. Each of these aspects of the planning process lends itself to climate risk analysis.
However, as discussed in Chapter 4, most utilities do not have regulatory guidance around assessing climate risks in planning or have siloed/uncoordinated processes that prevent a common understanding of priority climate risks — a necessary prerequisite for designing mitigation measures. Regulators can begin to address this issue by adding guidance to regulatory orders that identify what utilities should explicitly include in their filings to identify and assess climate risks and create clear instructions on how this assessment differs from separate resilience planning processes. In regions without appropriate publicly available climate data, such rulemaking activities will take time. Regulators can refer to Tables A and B for a starting point for a list of relevant risks, then ask utilities to assess the risks that are applicable to them, and provide justification for the ones that are not applicable. Table C in Chapter 4 provides a list of potential discovery questions with clear description of what the regulators and/or advocates should expect from the utility. In addition to dedicated sections in utility filings, regulators can create explicit expectations that utilities will face higher scrutiny if they cannot address certain risk assessment questions in their filing or response to the discovery questions. This creates an incentive for utilities to take a more proactive approach during planning to incorporate climate risks. Please refer to Chapter 5 for a complete discussion on how utilities can identify and assess climate risks. identify and assess climate risks.
2. Design new or leverage existing financial and regulatory incentives for utilities to transition away from fossil assets that present significantly increasing climate risks.
Fossil assets are associated with heightened physical and climate risks, creating major challenges for an affordability energy transition. Similarly, clean energy incentives display no clear connection to risk reduction. Financial and regulatory incentives can help drive utilities to proactively seek opportunities for clean energy investments, and regulators can take the following actions to create new incentives or make sure utilities are fully leveraging existing incentives:
- Require utilities to evaluate and leverage federal incentives to reduce costs and pass the savings benefits to customers.
Federal incentives, such as Energy Infrastructure Reinvestment (EIR) programs and tax credit community adders introduced in the Inflation Reduction Act (IRA), can create opportunities for clean energy redevelopment of legacy fossil sites, which produces net benefits for customers across the utility service territory — particularly in disadvantaged communities. These communities experience heightened vulnerability to climate change and extreme weather events on average.Fortunately, regulators can influence utility planning and investment decisions through many of the same venues. They can require utilities to include a section in their resource plans that detail how to leverage IRA incentives to benefit customers. They can also require utilities to establish a robust stakeholder engagement process to make sure all communities impacted by extreme weather events can participate in the conversations to evaluate investment decisions without over-indexing to any individual community.
- Create dedicated incentives for fuel cost risk management.
Electric utilities are authorized to get returns from capital-intensive investments and are typically authorized to pass 100 percent of fuel cost to customers. The fact that risks associated with fuel prices and price volatility are borne by customers creates a moral hazard and offers no incentive for the utility to manage those fuel costs. And since fuel prices are sensitive to climate risks (both physical and transition risks), better management of fuel costs and risk will mitigate associated climate risks.
Fuel cost recovery mechanisms can incentivize utility risk management. Some potential incentives include:
- Fuel-cost sharing: Companies bear part of the risk of fuel-cost volatility
- Fuel-cost true-up removal: The risk of fuel-price volatility is shifted back to utilities
- Fuel-risk reduction tariffs: Rate designs encourage utilities to better manage fuel costs and limit the risk to customers
- Planning and procurement: Process changes help reduce future fuel costs (e.g., all-source solicitation and procurement, fuel management plans, etc.)
- Strategies to increase access to information: Processes help inform regulator decisions about fuel costs (e.g., regular audits, enhanced prudence reviews, etc.)
- Efficiency ratio: A performance incentive mechanism rewards the utility for how efficiently it generates a megawatt-hour of power
Disallowance or the threat of disallowance can prevent utilities from recovering costs incurred due to uneconomic operation, which encourages utilities to manage fossil plants more actively.
3. Work with legislators and financial industry stakeholders to adequately and equitably distribute risks among taxpayers, shareholders, and ratepayers; particularly, explore ratepayer- or shareholder-backed self-insurance funds.
Regulators can reactively control costs associated with climate risks for LMI customers through energy affordability programs such as Percentage of Income Payment Plans (PIPPs). Still, there’s a broader need for regulators to work closely with state and federal legislators to explore alternative taxpayer funding opportunities to proactively distribute risks and costs. Similarly, regulators can collaborate with utilities as they engage their shareholders to explore innovative market mechanisms that efficiently transfer risks to the shareholders with the correct risk appetite.
Commercial insurance markets sometimes prove inefficient and overly expensive in managing the catastrophic risks introduced by low-probability, high-impact extreme events (e.g., wildfires and hurricanes). In these scenarios, utilities — and therefore ratepayers — might find it cheaper to bridge the gap by creating a fund for self-insurance. Of course, such funds generally require legislative backing or regulatory approval. California led the charge in this space by establishing the California Wildfire Fund in 2019 via AB 1054, which is designed to provide up to $21 billion in insurance protection for California’s three largest investor-owned utilities. Ratepayers and shareholders contribute equally to the fund. Other western states are beginning to follow suit. The Utah legislature recently passed SB224, which allows utilities to create a fire fund via ratepayer surcharge up to 50% of the utility’s Utah revenue requirement. This amounts to about $1 billion for Rocky Mountain Power.
Despite the benefits, self-insurance mechanisms place additional pressure on regulators to play an even more substantial role in reviewing the prudence of the process to make sure ratepayers don’t unfairly bear the burden of risks historically born by third parties in the commercial insurance market. Regulators can work with experts to carefully ensure utilities deploy a robust methodology to determine low-probability event risk — as part of a comprehensive risk assessment framework presented here — and set aside commensurate funds for self-insurance.
Chapter 7. Conclusion
A well-functioning risk mitigation strategy requires three main ingredients: an accurate and comprehensive assessment of overall risk, a responsive approach to mitigation measure design, and an equitable way of distributing the costs of both extreme event disaster response and mitigation measures. Many utility strategies currently lack one or more ingredients for climate risks. As the energy transition accelerates, and extreme weather spending along with it, regulators can take actions to ensure that utilities identify, assess, reduce, and distribute climate risks equitably — whether costs materialize retroactively or proactively. None of these steps can be implemented overnight and it may take time for the impact to be seen, but the earlier regulators and utilities take action, the more likely we can beat the speed of climate disasters while addressing reliability and affordability challenges.
Appendix 1: Methodology Overview of the Quantitative Example
Scenario data
For the quantitative example, we use wind, solar, and natural gas combined cycle (CC) data from the National Renewable Energy Laboratory (NREL) Electrification Futures Study (EFS). Specifically, we use aggregated existing natural gas numbers for capacity and generation from one EFS region for the gas scenario. We created the wind and solar scenario by splitting the capacity of the gas scenario between wind and solar while maintaining key normalized quantities (i.e., capacity factor, fixed cost per MW, and variable cost per MWh). As detailed in the EFS, future capacity factors of gas CC units are low and decrease over time. Therefore, the total generation in the wind and solar scenario is approximately 250% greater than that for the gas scenario. Since we are only using data at annual resolution all the substantial intra-annual variability in wind and solar resources are averaged out in our analysis. However, this work is focused on demonstrating the framework rather than carrying out a robust quantification of the climate risks in an individual region. When this framework is applied in practice, spatially and temporally consistent and granular data are essential.
Quantification of climate risks
In quantifying individual climate risks, we sought straightforward methods based on publicly available data or assumptions. For the impact of temperature on fuel cost we adopted the medium scenario assumption from In et al. — a 0.10% increase per year. We assume a carbon tax equal to $51/ton in 2020 dollars, which is the central estimate of the Interagency Working Group. Carbon capture and storage retrofit costs are included in the 2023 version of the NREL Annual Technology Baseline (ATB). We used ATB assumptions for estimating the total natural gas CC retrofit cost as well as increased operating costs. We further assumed that all natural gas CC units installed CCS technology to abate 90% of emissions in the year 2032. While these assumptions do account for inflation, they don’t account for the additional financing costs incurred by utilities when they build and depreciate capital assets over time, so our CCS estimate is likely conservative. For our natural gas price scenarios, we pulled daily Henry Hub spot prices and calculated annual medians for 2020-2022. We used these medians $1.90, $3.67, and $6.40 per MMBtu for the low, medium, and high fuel cost scenarios, respectively. To calculate fuel consumption from generation, we used the natural gas CC heat rate from the 2022 version of EIA-860. Finally, we used Lawrence Berkeley National Laboratory’s (LBNL) ReWEP tool to determine historical average wholesale prices for all ISOs. To remain temporally coincident with the natural gas price scenarios, we used median wholesale prices from 2020-2022 for the low, medium, and high wholesale price scenarios, which were $24.7, $46.6, and $67.8 per MWh, respectively. When inflation adjustments were performed (i.e., not inherited by the upstream data), we assumed inflation was 2.4%.
Appendix 2: Detailed Results from the Quantitative Example
Exhibit A1. Impact of higher air temperature on fuel cost
While modest, higher air temperatures reduce the efficiency of fossil plants, resulting in higher fuel consumption over time (Exhibit A1). This leads to fuel cost pass throughs to ratepayers gradually increasing over time.
Exhibit A2. Impact of carbon tax on total cost
Carbon taxes may be structured in several different ways. In any case, they increase the operating cost for fossil units (Exhibit A2), and the magnitude of the increase depends on the quantity of electricity produced by the plant as well as its marginal emissions rate. If treated similarly to other operating costs, these would be 100% rate-based, giving utilities an incentive to incur these costs rather than reduce them.
Exhibit A3. Impact of carbon capture retrofit costs on total gas unit costs
As shown in Exhibit A3, retrofitting a natural gas plant with CCS roughly equals the amount spent on OPEX over the modeling period while also increasing the OPEX marginally. Under the traditional cost-of-service regulation (COSR), utilities are encouraged to pursue such capital-intensive retrofit projects over other alternatives, which is known as the perverse incentive called capex bias. Some gas with CCS may provide an economically viable option as part of a net-zero portfolio, but utilities sometimes select gas with CCS over cleaner technologies, which likely results from outdated cost assumptions.
Exhibit A4. Impact of higher natural gas prices
Unlike retrofitting natural gas plants with CCS, volatile natural gas prices are often reflected in utility IRPs today. Since fuel costs are often passed through directly to customers, utilities have little to gain if fuel costs are higher than expected. However, underestimating natural gas price volatility could contribute to the narrative for explaining new gas over clean energy in preferred portfolios, so questioning natural gas price assumptions in IRPs remains a key role for regulators. The “Low” scenario in Figure A4, utilizes a 2020 baseline cost, showing the impact that a major event can have on natural gas demand and, in turn, prices. On the other end, the “High” scenario utilizes 2022 as the baseline, which coincides with Russia’s invasion of Ukraine. While it’s infeasible to predict such massive geopolitical or economic disruptions, the global system is exposed to a sufficient number of transition risks that utilities can expect more volatile prices in general and can modernize their approaches for forecasting price disruptions associated with extreme weather, which are expected to increase in both frequency and intensity in the coming years.
Exhibit A5. Impact of higher purchase costs
Regulated utilities purchase power from wholesale markets at vastly different fractions across the country, and purchased power is generally passed through directly to ratepayers. Naturally, a divide exists between those utilities that participate in a regional wholesale market with those that do not. However, wholesale prices are one of the best proxies available to determine how well a system as a whole is preparing for extreme weather events. Frequent extreme wholesale prices indicate poor performance and ideally incentivize additional generation to interconnect. All three scenarios shown in Exhibit A5 correspond to the same baseline years as the natural gas scenarios (i.e., 2020 for Low, 2021 for Medium, and 2022 for High), which illustrates the correlation between high natural gas prices and high wholesale purchase costs. In other words, the same geopolitical, economic, and extreme event disruptions are felt across both the natural gas and electric systems indicating an inability to hedge against price spikes in one with the other. This price correlation also highlights the importance of crafting scenarios using data aligned within a consistent time window.
Acknowledgements
Thanks to the Heising-Simons Foundation for their generous support of this insight brief. The authors thank the following individuals for graciously offering their insights to this work. Inclusion on this list does not indicate endorsement of the findings in the insight brief.
Rebecca O’Neil, PNNL
Maria Roumpani, Current Energy Group
Mark Specht, UCS
Soh Young In, KAIST
Diego Angel Hakim, Jacob Becker, Lauren Shwisberg, Joseph Daniel, RMI
Today’s grid infrastructure is underprepared for the changing trends in weather- and climate-related disasters. Between 2000-2023, extreme weather accounted for most major power outages in nearly every state. Likewise, utilities are not aligned with a transition to a net zero-carbon economy. Together, such physical climate risks (i.e., risks related to the physical impacts of climate change) and transition climate risks (i.e., risks related to the transition to a zero-carbon economy) threaten the affordability and reliability of clean electricity – the keystone of this future economy.
This insight brief explains how to integrate emerging climate risk assessment frameworks with utility planning processes to enable utilities to navigate a path toward a future grid supporting strong and resilient communities that can weather climate risks (Chapter 2). This process consists of four steps:
Step 1: Identify a list of climate risks and how each might impact the utility business.
Step 2: Assess the impact of climate risks on utility operation quantitatively.
Step 3: Mitigate both exposure to and impacts of climate risks on utility business.
Step 4: Distribute the risks across different stakeholders, including ratepayers and utility investors.
ES Exhibit 1. Utility Climate Risk Planning Process
Focusing on the first two steps, identification and assessment, this insight brief provides an overview of key physical (e.g., extreme heat and cold, drought, flooding, wildfire, hurricane, and rising average temperatures) and transition climate risks (e.g., carbon taxes, CCS retrofits, federal policy, falling technology costs, and emerging technologies) (Chapter 3), and lays out improvements to a climate risk assessment framework detailing how utilities can incorporate robust risk assessment into the planning processes (Chapter 4), with three main components:
- Covers the full range of physical and transition climate risks. It is critical to ensure utilities assess all the risks relevant in a utility service area rather than selecting only the ones that are in favor of their preferred portfolio.
- Defines the methodology for quantifying each risk starting at the asset level and aggregating up to the portfolio level. Specifically, two key datasets — an hourly multi-year utility-specific weather dataset to capture impacts on the system on operational timelines and a 30+ year utility-specific climate dataset to help plan for the chronic realities and extreme events of the future — are critical to ensuring a robust assessment of risks and interannual variability in the modeling efforts.
- Specifies exactly how and where each quantified risk feeds into utility planning models.
Regulators (and other stakeholders working closely with regulators in utility proceedings) are encouraged to consider a suite of actions to ensure utilities take the necessary steps to incorporate climate risks into planning and drive conversations around the trade-offs of risk mitigation versus acceptance in the transition:
- Add climate risk identification and assessment requirements to planning processes.
- Create or leverage existing financial and regulatory incentives for utilities to transition away from fossil assets that present significantly increasing climate risks.
- Work with legislators and financial industry stakeholders to adequately and equitably distribute climate risks among taxpayers, shareholders, and ratepayers, particularly, explore ratepayer- or shareholder-backed self-insurance funds.
As the energy transition accelerates, and extreme weather-related spending along with it, there’s an imperative for regulators and other stakeholders to work with utilities to align on a streamlined and proactive process of identifying, assessing, reducing and distributing climate risks, which create opportunities for a reliable and equitable future.
Utility spending in response to weather- and climate-related forces continues to increase. Extreme weather events including hurricanes, wildfires, extended heat domes, and winter storms as well as shifting climate patterns, such as precipitation, put strain on our aging grid infrastructure. Simultaneously, global market trends further increase supply uncertainty and exacerbate cost volatility in the utility business. Evolving regional regulations introduce opportunities for more effective climate mitigation while also creating challenges for maintaining stability and predictability in long-term planning. Collectively, these factors can increase the cost of running the electric grid, which trickles down to customers’ electricity bills, creating affordability challenges and disproportionately affecting those who live in disadvantaged communities. In California, three major investor-owned utilities have seen wildfire-related costs grow from 2% of the revenue requirement in 2019 to 16% in 2022, and PG&E recently experienced an even more drastic increase in wildfire-related costs — 117% between January 2023 and April 2024, contributing to 24% of the total system revenue requirement as of April 2024.
Effective utility planning can provide the opportunity to manage climate risks if utilities make investment decisions that are informed by a comprehensive risk assessment, but utilities and their regulators must see eye-to-eye to move successfully from risk assessment to risk mitigation. Not every climate resilience measure provides a net cost benefit, which highlights the importance of regulatory prudence reviews. This insight brief presents an overview of climate risks, examines the shortcomings of current utility planning processes, and lays out solutions to improve the analytical framework for assessing climate risks. Implemented successfully, these updates can facilitate climate risk mitigation while achieving reliable and equitable decarbonization of the electric power system.
Chapter 2. Recommended Steps for Utility Climate Risk Planning
Existing utility risk assessment and mitigation processes could accommodate the addition of climate risks. The US Department of Energy and the Electric Power Research Institute (EPRI)’s Climate Resilience and Adaptation Initiative (Climate READi) lay out best practices for vulnerability assessment and resilience planning and for representing climate impacts in power system models, respectively. Exhibit 1 provides a simplified view of typical steps that show up in these and other emerging utility climate risk assessment and planning frameworks. However, we explicitly separate a step focused on distributing climate risk and overlay implicated regulatory venues, which can help findings from assessments impact utility business decisions. Finally, risk assessment processes possess a cyclical nature, with modeling assumptions, progress, and new information continuously informing the next iteration of the process.
Exhibit 1. Utility Climate Risk Planning Process

Briefly, integrating climate risk into integrated resource plans (IRPs), distribution system plans (DSPs), rate cases, and other utility planning processes comprises four main steps:
Step 1: Identify the scope of the analysis (i.e., what planning processes and modeling exercises are implicated), develop a comprehensive set of regionally relevant climate risks, collect necessary data, and determine how each risk might impact the utility business. This can occur during the resource and grid infrastructure planning processes (IRPs, DSPs, risk-specific plans such as wildfire mitigation plans) as well as inform operational decisions for existing assets.
Step 2: Assess the likelihood and severity of impacts stemming from climate risk on the utility business and customers quantitatively. This can occur during the same processes as in Step 1.
Step 3: Mitigate both exposure to and possible impacts associated with climate risks on the utility business using a portfolio of climate resilience methods. We summarize risk reduction actions in two main groups:
- Avoid exposure to climate risks where practical. For example, switching to renewable portfolios avoids exposure to fuel cost volatility.
- Reduce the impacts of climate risks where they cannot be avoided or where avoidance is cost prohibitive. For example, weatherizing power plants reduces the likelihood of frozen power plant components during winter storms.
Risk mitigation actions may occur in a variety of utility planning processes. Resource and grid infrastructure planning processes (IRPs, DSPs, risk-specific plans such as wildfire mitigation plans) offer the most direct venue for implementing climate risk mitigation measures such as generation portfolio shifts and grid hardening. Rate cases, performance-based regulation (PBR) dockets, and fuel recovery dockets also offer opportunities for risk mitigation by creating incentives for utilities to transition away from fossil fuel assets and invest in grid resilience improvement.
Step 4: Distribute the risks across different stakeholders, including ratepayers, utility investors, etc. There are two strategies for risk distribution:
- Reactive measures: After risks materialize and incur costs (e.g., fuel volatility or wildfires increase expenses), stakeholders can explore risk distribution approaches by proposing mechanisms for equitable cost allocation, particularly considering ones that alleviate the energy burden for low- and middle-income (LMI) customers and thereby preventing climate risks from producing a significant affordability crisis in disadvantaged communities. This may occur during rate cases, PBR dockets, fuel cost recovery dockets, etc.
- Proactive measures: Before the risks materialize, stakeholders can alter resource planning rules and incentive structures (e.g., fuel cost sharing) to prevent risks from materializing, balance risks among ratepayers, utilities, and investors, or transfer risks to third parties (e.g., insurance companies). This may occur in traditional resource and grid planning processes (IRPs, DSPs, risk-specific plans such as wildfire mitigation plans) or within the same regulatory venues as the reactive process (i.e., rate cases, PBR dockets, etc.).
While existing climate risk mitigation frameworks imply some distribution of risk within the risk mitigation step, we separate it here to highlight regulators’ central role in this step and point out the additional regulatory venues where risk mitigation action can take place.
This insight brief primarily focuses on Steps 1 and 2 detailing how to identify and assess climate risks. Steps 3 and 4 are discussed briefly in the later sections, with further research needs flagged for future projects.
Chapter 3. Identifying Climate Risks
Climate change poses financial risks to the utility business. Climate related risks fall broadly into two categories: physical risks and transition risks. The Task Force on Climate-related Financial Disclosures (TCFD) provides industry standard recommendations for disclosing climate risks falling into both categories, which have been widely adopted across companies, including some US electric utilities. The US Securities and Exchange Commission (SEC) integrated ideas from the TCFD and the GHG Protocol into their proposed climate risk disclosure requirement, which may become mandatory for public companies in the United States. Given repeated exposure to climate risks and their obligation to serve customers, disclosure is just a starting point for the utility industry.
Physical Climate Risks
Physical climate risks refer to those associated with physical impacts from climate change. Such risks may manifest as acute extreme weather events (such as historic floods, heat waves, and winter storms), or as chronic conditions that represent a new normal (such as rising average temperatures, rising sea levels, and shifting precipitation patterns). These risks can lead to more frequent reduction or disruption in the power supply, impacting reliability and resilience. Further complicating matters, some of these risks are correlated – resulting in events occurring simultaneously or in close succession that result in greater impacts.
Physical climate risks affect all customers, but the impacts of these risks differ among customers. For example, more than 25 million Americans in the Justice40 communities —which are defined as “disadvantaged communities that are marginalized by underinvestment and overburdened by pollution” – are expected to face at least 48 health-threatening heat days per year by 2050. The customers in these underserved communities, who sometimes experience affordability problems during normal days, can face increasingly difficult decisions during extreme weather events as they are driven to restrict their energy use (e.g., limit use of air conditioning during extreme heat), out of fear of unaffordable utility bills. Table A below provides an illustrative sample of the physical climate risks and possible impacts.
Table A. Descriptions of Physical Climate Risks
Transition Climate Risks
Transition climate risks are related to the impending transition to a lower carbon economy, or put differently, risks that laggards will assume by waiting or refusing to take action on the energy transition (e.g., utilities building new gas infrastructure without a plan to switch fuels or including CCS costs in planning models). Transition risks can be broken down into different categories: policy, legal, technological, market, and reputational, all of which are associated with the directive to reduce greenhouse gas emissions and transition to clean energy.
Federal policies such as the Inflation Reduction Act and Infrastructure Investment and Jobs Act have created incentives for zero-carbon technologies. On the other hand, load growth driven by datacenters and advanced manufacturing facilities top the list of drivers behind utilities’ proposals to build new gas plants, creating challenges to meet decarbonization targets set by the utility companies and state legislation. In the meantime, new or revised environmental regulations — such as the Environmental Protection Agency’s (EPA) power plant emission rules — have potentially significant impact on a utility’s operational costs. All these risks contribute to significant uncertainty in the financial impact of utility planning and investment pathways, and if not managed well, these risks may translate into unexpected bill increases. Table B below provides an illustrative sample of the transition climate risks and possible impacts.
These tables are not designed to act as an exhaustive list of all the risks that can possibly impact certain jurisdictions, and each state/utility is expected to conduct a customized assessment to identify the risks that are applicable to the local context. However, it can be a starting point for the regulators and advocates to request utilities to provide evidence around how certain risks may or may not impact their jurisdictions.
Table B. Descriptions of Transition Climate Risks
Chapter 4. Current Practices and Challenges in Climate Risk Assessments
Utilities are starting to explore practices that assess the exposure to climate risks in collaboration with industry experts. EPRI has started to consolidate resources under the Climate READi framework, and independent consultants (e.g., ICF) have started to provide climate risk assessment services to major utilities. Furthermore, some utilities are beginning to assess a subset of climate risks in IRPs, DSPs, or in dedicated risk-specific plans (e.g., wildfire mitigation plans). New York’s ConEd, Southern California Edison, Chicago’s ComEd, and Seattle City Light are leading the way with their climate plans.
Seattle City Light Climate Change Vulnerability Assessment and Adaptation Plan
In 2013, Seattle City Light’s strategic plan established objectives to research the impacts of climate change on the utility and develop an adaptation plan centered around specific mitigation actions. The plan maps eight physical climate hazards to possible impacts to five aspects of utility infrastructure resulting in thirteen total impact pathways. Finally, the report summarized adaptation actions for each aspect of utility infrastructure. While not mandated by any legislative or regulatory body, this plan benefitted from its forward thinking and the detailed description of potential actions. However, it lacks mechanisms for monitoring and enforcement.
While these efforts lay a solid foundation for establishing best practices in climate risk assessment within the planning process, utilities still face challenges in integration and data accessibility, impeding a seamless and comprehensive approach to climate-informed decision-making. For example, separate analyses are usually conducted for each utility modeling exercise (e.g., wind and solar resource forecasts, distributed energy plans, load forecasts, natural gas pipeline expansion and maintenance schedules, etc.) , and the audience and time horizon often differ among these analyses. Furthermore, access to regionally relevant high-resolution climate data and expertise to use this data correctly remains a challenge. This stems from the fact that historical weather data fails to adequately represent future climate risks and access to climate data remains uneven among utilities. Multiple organizations are actively working on solutions to address this data asymmetry – EPRI’s Climate READi and Argonne National Laboratory’s Climate Risk and Resilience (ClimRR) data portal.
Some utilities have multiple planning proceedings, with one or more dedicated to climate risk assessment, often referred to as climate resilience plans, grid security plans, etc. While this represents a solid first step, a siloed process addressing the impacts of climate change introduces additional overhead potentially increasing the difficulty of implementing findings in existing planning and operational processes.
Duke’s Carbon Plan in North Carolina
In September 2023, Duke Energy Carolinas and Duke Energy Progress released a report that provides an assessment of the climate resilience impact of its grid infrastructure investments performed by ICF. However, it focuses only on the physical risk impact of climate change (e.g., high temperature and extreme heat, extreme cold and ice, etc.) without covering transition risks, and it remains unclear what mechanism will encourage Duke to implement any of the recommendations from the analysis. Separately, Duke has been pursuing its resource planning effort through the Carbon Plan proceedings, and although their assessment of forthcoming EPA rules indicated substantial cost impacts, Duke failed to include a discussion around the impact of alternative portfolios.
Without strong regulatory guidance ahead of planning processes, utilities do not have a concrete baseline to move towards risk mitigation and allocation. When a reactive approach is taken after these risks materialize, they translate into costs for ratepayers, resulting in rising bills and stifling energy burdens for LMI customers. In the past few months, PacifiCorp filed multiple requests in several of its jurisdictions to seek deferral of the wildfire-related insurance costs and recover significant insurance premiums. These costs contribute to a portion of the request for a 26.8% rate hike for PacifiCorp’s customers in Idaho and 28.3% increase for customers in Utah, which will further elevate the energy burden in these states. Proactive measures can offer a win-win where they provide a net cost benefit or shift risk away from LMI customers.
Chapter 5. Improving Climate Risk Assessments
This section introduces additional factors that can be deployed by utilities to help better quantify climate risks and assess the distribution of climate risks across different ratepayer classes. Regulators and advocates are likely not equipped with the data and modeling resources to conduct their own analysis that deploys this framework, but they are well positioned to play a critical role in nudging the utilities to deploy or improve climate risk assessments by requesting the utilities to provide information on their analytical approaches and modeling processes. The requirements laid out by the California Public Utilities Commission can offer a starting place for other states considering climate risk assessments. The section “Questions for Regulators and Advocates on Climate Risk Planning” includes a list of targeted questions for regulators and advocates to pose in regulatory proceedings.
A climate risk assessment framework includes the following components:
- Covers the full range of physical and transition climate risks. It is critical to ensure utilities assess all the risks relevant in a utility service area rather than selecting only the ones that are in favor of their preferred portfolio. Physical climate risk identification and assessment requires expert knowledge of weather and climate science as well as how climate events can impact utility assets, whereas transition climate risk assessment requires expert knowledge of policy, economics, and finance. Weather and climate science is new territory for most utilities, so they should engage these new experts in their planning processes.
- Defines the methodology for quantifying each risk starting at the asset level and aggregating up to the portfolio level. When viewed in isolation or for an individual asset, some risks may appear manageable, but if multiple risks materialize at the same time, in quick succession, or cover a large enough part of the portfolio, the picture may change. Core to these methodologies lie two datasets:
- An hourly multi-year utility-specific weather dataset that captures a reasonable distribution of future conditions, which affect the system on operational timelines. Ideally, this dataset combines high-quality historical observational data products and high-resolution downscaled climate data. This dataset acts as the foundation for all load forecasting and production cost modeling runs.
- A 30+ year utility-specific climate dataset that captures the distributions of future temperature, precipitation, wind, and humidity to help plan for the chronic realities and extreme events of the future. This dataset acts as the foundation for long-term planning model runs, and the long-time horizon is required to capture variability across scales (e.g., extreme events, year-to-year variability, El Niño Southern Oscillation (ENSO) intensity, drought patterns, etc.). ENSO provides a key example for the necessity of a long-time horizon in climate datasets: it cycles irregularly every 2-7 years, and its intensity changes on a 10-15 year timeline. Therefore, a dataset spanning less than 7 years may only capture one aspect of the ENSO cycle and any dataset shorter than 15 years may only capture a single level of intensity. Furthermore, a 30-year dataset is hardly adequate for capturing extreme events — containing at best a 1-in-30-year event. While this meets the 1-in-10-year criteria applied in many resource adequacy studies, it fails to capture the 100-year events experienced by so many utilities in the past several years. In other words, extreme event modeling in particular requires a dataset much longer than those traditionally used in utility planning, but is necessary to adequately characterize the range of conditions that proposed 20–50 year assets will experience between today and 2075.
- Specifies exactly how and where each quantified risk feeds into utility planning models. For example, load forecasting, production cost modeling, and capacity expansion modeling for IRPs, DSPs, etc. It can also define a strategy for how the decision-making process addressing uncertainty.
Exhibit 2 below provides a conceptual illustration of how an improved climate risk assessment might affect portfolio selection in an IRP. In the scenario where no climate risk is considered (the “Base Scenario Cost” bar), capacity expansion modeling may produce a fossil-heavy portfolio that’s financially competitive compared to a clean energy portfolio. By integrating a climate risk assessment with capacity expansion and production cost modeling, planners can estimate previously externalized costs (the “Climate Risk Impact” bar), which may result in a lower overall cost for the clean energy portfolio.
Exhibit 2. Illustrative Risk Assessment Comparison
A large fraction of financial risks come from market uncertainties and fluctuating operating expenses, which are costs that are mostly classified as energy-related (i.e., variable) costs. Energy-related costs have a disproportionate impact on residential customers because, most often, the largest share of energy-related costs is allocated to the residential class in the cost allocation process. Additionally, commercial and industrial customers, who pay a larger fraction of demand-related costs through demand charges, have accessibility to hedging and direct procurement opportunities, which insulate them from the impact of high energy-related costs. In other words, clean energy portfolios – much less prone to market fluctuation due to fuel cost uncertainties and overall market uncertainties once they are in service – create more robust benefits for residential customers, including the ones that live in disadvantaged communities.
Quantitative Example of Deploying an Improved Modeling Framework
To demonstrate how this improved modeling framework works, we present an example that captures a subset of diverse climate risks and how their impacts are layered upon one another, creating a more expensive future compared to a simple baseline cost assumption. In this example, we create two sample portfolios: The gas scenario builds new gas resources, and the renewable scenario builds equal parts wind and solar with the same capacity as the gas resources. We assume that the rest of the portfolio is identical between the two scenarios, and therefore exclude it for brevity. Exhibit 3 below illustrates the ratepayer cost savings in the renewable scenario relative to the gas scenario, when both scenarios are exposed to a set of climate risks.Exhibit 3. Total Climate Risk Assessment Cost Comparison
Digging into the possible impact of each risk, CCS retrofits, carbon taxes, and natural gas prices stand out as the largest drivers of cost increase in the gas scenario, highlighting the uncertainty surrounding tightening carbon regulations and EPA rules. Carbon taxes or regulations are often qualitatively discussed or quantitatively assessed in sensitivity scenarios that do not directly inform the resource portfolio decision. Volatile natural gas prices and purchase cost increases, on the other hand, are often reflected in the IRP modeling, but utilities systematically underestimate the impact of extreme weather events on gas prices and purchase costs, leading to an insufficient picture of these risks in preferred portfolio resource modeling. We used moderate assumptions in this example, yet the gas scenario costs exceed the renewables scenario costs by 68%. In a complete implementation, each risk would ideally be represented by a probabilistic distribution. Methodological details underlying this example are provided in Appendix 1 and more detailed results are provided in Appendix 2.
Questions for Regulators and Advocates on Climate Risk Planning
The questions that we lay out in this section represent a starting point to help regulators investigate a utility’s process for identifying and assessing climate risks but are by no means exhaustive.
Table C. Discovery Questions for Regulators and Advocates
PUC Review Expectation
Discovery Questions
High-level Consideration: How is the utility accounting for physical climate risks during planning?
Utility employs an hourly multi-year utility-specific weather dataset.
- Please describe the historical weather data used in all modeling activities including the spatial and temporal resolution, the geographic coverage, and the time horizon.
- If the dataset’s temporal resolution is lower than hourly, explain what steps you’ve taken to capture the relevant intraday variability in all meteorological variables.
- If the dataset covers only a single year, explain what steps you’ve taken to account for the full range of past extreme events.
- If the temporal resolution is greater than 2km, explain what steps you’ve taken to correct for terrain effects lost at lower resolutions.
Utility employs a 30+ year utility-specific climate dataset.
- Please describe your process for downscaling climate data to produce a relevant dataset for your jurisdiction (i.e., what climate data product(s) are you using, is your process statistical or dynamical, what are the final spatial and temporal resolution, the geographic coverage, and the time horizon of the resulting dataset).
- Describe what combination of historical and projected data you use in load forecasting, capacity expansion, and projection cost modeling activities. If you are not using any projected data, explain how you’re capturing future conditions. If you are using projected data, explain the process by which you projected that data and the relationship between this data and your downscaled climate data.
Utility performs an assessment of all relevant physical climate risks.
- Provide statistics covering the frequency and severity of all relevant extreme weather events (e.g., heat waves, winter storms, flooding, hurricanes, or wildfires) over the entire horizon of your planning processes. If both the frequency and severity are not increasing over long time horizons, justify this trend using evidence from your climate dataset.
Utility load forecast is built upon the weather and climate datasets.
- Describe exactly how your weather and climate datasets impact your load forecast. Does your load forecast account for changing technology adoption trends (e.g., air conditioning, heat pumps, air purifiers, etc.) stemming from a gradually warming world? If not, show why using evidence from your climate dataset.
High-level Consideration: How is the utility accounting for transition climate risks during planning?
Utility performs an assessment of all relevant transition climate risks.
- Discuss individually which policy and regulatory, legal, technological, operational, and market risks you’re tracking. If you’re not tracking any risks in a certain category provide a justification.
Utility performs an assessment of all relevant policy and regulatory risks.
- Provide a detailed list of individual policies – at both the state and federal levels – that you’ve included in long-term planning.
- Did you model any type of carbon price? If so, provide explanations for the range of prices used in modeling. If you only included a carbon price in some scenarios, explain your reasoning.
Utility financial forecast includes spending on climate- and weather-related litigation.
- Provide a full list of past climate- and weather-related litigation claims, and report how much litigation-related spending appears in your forecast.
- If your forecast doesn’t show increased spending on climate- and weather-related litigation on a forward-looking basis, explain what mitigation efforts you’ve completed or planned to reduce such liabilities and their associated costs.
Utility performs technology cost sensitivity analyses.
- Explain your methodology for forecasting capital and operational expenditures for new units particularly the origin of your assumptions around clean technologies with rapidly decreasing prices (e.g., storage, solar, or wind) and emerging clean firm technologies with highly uncertain prices (e.g., long-duration storage, small modular reactors, or advanced geothermal).
Utility performs an assessment of the transition strategy of new or existing fossil resources.
- If an IRP includes continued operation of existing fossil assets or proposed new gas, provide a detailed description of your plan to comply with future rules limiting carbon dioxide emissions for both new and existing plants.
- If the plan involves fuel-switching to hydrogen, include plans and costs associated with delivering hydrogen to the proposed natural gas facility including the proposed source of the hydrogen.
- If the plan involves CCS, include plans and costs associated with transporting and sequestering CO2 produced by the proposed natural gas facility. These transport costs should differ based on the distance to the long-term CO2 sequestration facility.
- If the plan involves CCS, describe in detail locations and capacities of long-term CO2 sequestration facilities. If you plan to use a third-party off taker, report their current offtake capacity as a percentage of the amount you expect them to offtake from your power plants.
Utility performs an assessment of all relevant market risks.
- Explain quantitatively how you forecast natural gas prices over the planning horizon.
- If the price forecast is single-valued (i.e., not probabilistic), provide a justification for neglecting the uncertainty inherent in the price forecast.
- Would a persistently higher natural gas price result in different resource builds or accelerated retirements compared to your current/preferred portfolio?
- Explain qualitatively and quantitatively how you account for natural gas supply disruptions in your planning models.
- Explain how costs incurred during short-run (i.e., weather-driven) supply disruptions and long-run (i.e., geopolitics-driven) supply disruptions would impact cash flows and ratepayer bills.
- Explain quantitatively how you forecast wholesale electricity prices over the planning horizon.
- If the price forecast is single-valued (i.e., not probabilistic), provide a justification for neglecting the uncertainty inherent in the price forecast.
- Would persistently higher wholesale electricity prices result in different resource builds compared to your current/preferred portfolio?
Chapter 6. What’s Next: What Regulators Can Do to Address Climate Risks
The improvements discussed above can help utilities gain a better understanding of what climate risks exist, how they manifest in operations, and how to quantify potential impacts during the planning process. However, in no jurisdiction has a comprehensive framework, as outlined in this paper, been implemented. Fortunately, regulators and policymakers may have solutions at their disposal to address any potential gaps:
1. Require climate risk identification and assessment requirements to planning processes.
One of the best opportunities for a utility to identify climate risks and take steps to avoid or reduce those risks are forward-looking planning proceedings, including resource and distribution planning. Where these processes are already mandated, they typically require utilities to conduct load forecasting, commodity price forecasting, and are a platform by which the utility makes decision on resources. Each of these aspects of the planning process lends itself to climate risk analysis.
However, as discussed in Chapter 4, most utilities do not have regulatory guidance around assessing climate risks in planning or have siloed/uncoordinated processes that prevent a common understanding of priority climate risks — a necessary prerequisite for designing mitigation measures. Regulators can begin to address this issue by adding guidance to regulatory orders that identify what utilities should explicitly include in their filings to identify and assess climate risks and create clear instructions on how this assessment differs from separate resilience planning processes. In regions without appropriate publicly available climate data, such rulemaking activities will take time. Regulators can refer to Tables A and B for a starting point for a list of relevant risks, then ask utilities to assess the risks that are applicable to them, and provide justification for the ones that are not applicable. Table C in Chapter 4 provides a list of potential discovery questions with clear description of what the regulators and/or advocates should expect from the utility. In addition to dedicated sections in utility filings, regulators can create explicit expectations that utilities will face higher scrutiny if they cannot address certain risk assessment questions in their filing or response to the discovery questions. This creates an incentive for utilities to take a more proactive approach during planning to incorporate climate risks. Please refer to Chapter 5 for a complete discussion on how utilities can identify and assess climate risks. identify and assess climate risks.
2. Design new or leverage existing financial and regulatory incentives for utilities to transition away from fossil assets that present significantly increasing climate risks.
Fossil assets are associated with heightened physical and climate risks, creating major challenges for an affordability energy transition. Similarly, clean energy incentives display no clear connection to risk reduction. Financial and regulatory incentives can help drive utilities to proactively seek opportunities for clean energy investments, and regulators can take the following actions to create new incentives or make sure utilities are fully leveraging existing incentives:
- Require utilities to evaluate and leverage federal incentives to reduce costs and pass the savings benefits to customers.
Federal incentives, such as Energy Infrastructure Reinvestment (EIR) programs and tax credit community adders introduced in the Inflation Reduction Act (IRA), can create opportunities for clean energy redevelopment of legacy fossil sites, which produces net benefits for customers across the utility service territory — particularly in disadvantaged communities. These communities experience heightened vulnerability to climate change and extreme weather events on average.Fortunately, regulators can influence utility planning and investment decisions through many of the same venues. They can require utilities to include a section in their resource plans that detail how to leverage IRA incentives to benefit customers. They can also require utilities to establish a robust stakeholder engagement process to make sure all communities impacted by extreme weather events can participate in the conversations to evaluate investment decisions without over-indexing to any individual community.
- Create dedicated incentives for fuel cost risk management.
Electric utilities are authorized to get returns from capital-intensive investments and are typically authorized to pass 100 percent of fuel cost to customers. The fact that risks associated with fuel prices and price volatility are borne by customers creates a moral hazard and offers no incentive for the utility to manage those fuel costs. And since fuel prices are sensitive to climate risks (both physical and transition risks), better management of fuel costs and risk will mitigate associated climate risks.
Fuel cost recovery mechanisms can incentivize utility risk management. Some potential incentives include:
- Fuel-cost sharing: Companies bear part of the risk of fuel-cost volatility
- Fuel-cost true-up removal: The risk of fuel-price volatility is shifted back to utilities
- Fuel-risk reduction tariffs: Rate designs encourage utilities to better manage fuel costs and limit the risk to customers
- Planning and procurement: Process changes help reduce future fuel costs (e.g., all-source solicitation and procurement, fuel management plans, etc.)
- Strategies to increase access to information: Processes help inform regulator decisions about fuel costs (e.g., regular audits, enhanced prudence reviews, etc.)
- Efficiency ratio: A performance incentive mechanism rewards the utility for how efficiently it generates a megawatt-hour of power
Disallowance or the threat of disallowance can prevent utilities from recovering costs incurred due to uneconomic operation, which encourages utilities to manage fossil plants more actively.
3. Work with legislators and financial industry stakeholders to adequately and equitably distribute risks among taxpayers, shareholders, and ratepayers; particularly, explore ratepayer- or shareholder-backed self-insurance funds.
Regulators can reactively control costs associated with climate risks for LMI customers through energy affordability programs such as Percentage of Income Payment Plans (PIPPs). Still, there’s a broader need for regulators to work closely with state and federal legislators to explore alternative taxpayer funding opportunities to proactively distribute risks and costs. Similarly, regulators can collaborate with utilities as they engage their shareholders to explore innovative market mechanisms that efficiently transfer risks to the shareholders with the correct risk appetite.
Commercial insurance markets sometimes prove inefficient and overly expensive in managing the catastrophic risks introduced by low-probability, high-impact extreme events (e.g., wildfires and hurricanes). In these scenarios, utilities — and therefore ratepayers — might find it cheaper to bridge the gap by creating a fund for self-insurance. Of course, such funds generally require legislative backing or regulatory approval. California led the charge in this space by establishing the California Wildfire Fund in 2019 via AB 1054, which is designed to provide up to $21 billion in insurance protection for California’s three largest investor-owned utilities. Ratepayers and shareholders contribute equally to the fund. Other western states are beginning to follow suit. The Utah legislature recently passed SB224, which allows utilities to create a fire fund via ratepayer surcharge up to 50% of the utility’s Utah revenue requirement. This amounts to about $1 billion for Rocky Mountain Power.
Despite the benefits, self-insurance mechanisms place additional pressure on regulators to play an even more substantial role in reviewing the prudence of the process to make sure ratepayers don’t unfairly bear the burden of risks historically born by third parties in the commercial insurance market. Regulators can work with experts to carefully ensure utilities deploy a robust methodology to determine low-probability event risk — as part of a comprehensive risk assessment framework presented here — and set aside commensurate funds for self-insurance.
Chapter 7. Conclusion
A well-functioning risk mitigation strategy requires three main ingredients: an accurate and comprehensive assessment of overall risk, a responsive approach to mitigation measure design, and an equitable way of distributing the costs of both extreme event disaster response and mitigation measures. Many utility strategies currently lack one or more ingredients for climate risks. As the energy transition accelerates, and extreme weather spending along with it, regulators can take actions to ensure that utilities identify, assess, reduce, and distribute climate risks equitably — whether costs materialize retroactively or proactively. None of these steps can be implemented overnight and it may take time for the impact to be seen, but the earlier regulators and utilities take action, the more likely we can beat the speed of climate disasters while addressing reliability and affordability challenges.
Appendix 1: Methodology Overview of the Quantitative Example
Scenario data
For the quantitative example, we use wind, solar, and natural gas combined cycle (CC) data from the National Renewable Energy Laboratory (NREL) Electrification Futures Study (EFS). Specifically, we use aggregated existing natural gas numbers for capacity and generation from one EFS region for the gas scenario. We created the wind and solar scenario by splitting the capacity of the gas scenario between wind and solar while maintaining key normalized quantities (i.e., capacity factor, fixed cost per MW, and variable cost per MWh). As detailed in the EFS, future capacity factors of gas CC units are low and decrease over time. Therefore, the total generation in the wind and solar scenario is approximately 250% greater than that for the gas scenario. Since we are only using data at annual resolution all the substantial intra-annual variability in wind and solar resources are averaged out in our analysis. However, this work is focused on demonstrating the framework rather than carrying out a robust quantification of the climate risks in an individual region. When this framework is applied in practice, spatially and temporally consistent and granular data are essential.
Quantification of climate risks
In quantifying individual climate risks, we sought straightforward methods based on publicly available data or assumptions. For the impact of temperature on fuel cost we adopted the medium scenario assumption from In et al. — a 0.10% increase per year. We assume a carbon tax equal to $51/ton in 2020 dollars, which is the central estimate of the Interagency Working Group. Carbon capture and storage retrofit costs are included in the 2023 version of the NREL Annual Technology Baseline (ATB). We used ATB assumptions for estimating the total natural gas CC retrofit cost as well as increased operating costs. We further assumed that all natural gas CC units installed CCS technology to abate 90% of emissions in the year 2032. While these assumptions do account for inflation, they don’t account for the additional financing costs incurred by utilities when they build and depreciate capital assets over time, so our CCS estimate is likely conservative. For our natural gas price scenarios, we pulled daily Henry Hub spot prices and calculated annual medians for 2020-2022. We used these medians $1.90, $3.67, and $6.40 per MMBtu for the low, medium, and high fuel cost scenarios, respectively. To calculate fuel consumption from generation, we used the natural gas CC heat rate from the 2022 version of EIA-860. Finally, we used Lawrence Berkeley National Laboratory’s (LBNL) ReWEP tool to determine historical average wholesale prices for all ISOs. To remain temporally coincident with the natural gas price scenarios, we used median wholesale prices from 2020-2022 for the low, medium, and high wholesale price scenarios, which were $24.7, $46.6, and $67.8 per MWh, respectively. When inflation adjustments were performed (i.e., not inherited by the upstream data), we assumed inflation was 2.4%.
Appendix 2: Detailed Results from the Quantitative Example
Exhibit A1. Impact of higher air temperature on fuel cost
While modest, higher air temperatures reduce the efficiency of fossil plants, resulting in higher fuel consumption over time (Exhibit A1). This leads to fuel cost pass throughs to ratepayers gradually increasing over time.
Exhibit A2. Impact of carbon tax on total cost
Carbon taxes may be structured in several different ways. In any case, they increase the operating cost for fossil units (Exhibit A2), and the magnitude of the increase depends on the quantity of electricity produced by the plant as well as its marginal emissions rate. If treated similarly to other operating costs, these would be 100% rate-based, giving utilities an incentive to incur these costs rather than reduce them.
Exhibit A3. Impact of carbon capture retrofit costs on total gas unit costs
As shown in Exhibit A3, retrofitting a natural gas plant with CCS roughly equals the amount spent on OPEX over the modeling period while also increasing the OPEX marginally. Under the traditional cost-of-service regulation (COSR), utilities are encouraged to pursue such capital-intensive retrofit projects over other alternatives, which is known as the perverse incentive called capex bias. Some gas with CCS may provide an economically viable option as part of a net-zero portfolio, but utilities sometimes select gas with CCS over cleaner technologies, which likely results from outdated cost assumptions.
Exhibit A4. Impact of higher natural gas prices
Unlike retrofitting natural gas plants with CCS, volatile natural gas prices are often reflected in utility IRPs today. Since fuel costs are often passed through directly to customers, utilities have little to gain if fuel costs are higher than expected. However, underestimating natural gas price volatility could contribute to the narrative for explaining new gas over clean energy in preferred portfolios, so questioning natural gas price assumptions in IRPs remains a key role for regulators. The “Low” scenario in Figure A4, utilizes a 2020 baseline cost, showing the impact that a major event can have on natural gas demand and, in turn, prices. On the other end, the “High” scenario utilizes 2022 as the baseline, which coincides with Russia’s invasion of Ukraine. While it’s infeasible to predict such massive geopolitical or economic disruptions, the global system is exposed to a sufficient number of transition risks that utilities can expect more volatile prices in general and can modernize their approaches for forecasting price disruptions associated with extreme weather, which are expected to increase in both frequency and intensity in the coming years.
Exhibit A5. Impact of higher purchase costs
Regulated utilities purchase power from wholesale markets at vastly different fractions across the country, and purchased power is generally passed through directly to ratepayers. Naturally, a divide exists between those utilities that participate in a regional wholesale market with those that do not. However, wholesale prices are one of the best proxies available to determine how well a system as a whole is preparing for extreme weather events. Frequent extreme wholesale prices indicate poor performance and ideally incentivize additional generation to interconnect. All three scenarios shown in Exhibit A5 correspond to the same baseline years as the natural gas scenarios (i.e., 2020 for Low, 2021 for Medium, and 2022 for High), which illustrates the correlation between high natural gas prices and high wholesale purchase costs. In other words, the same geopolitical, economic, and extreme event disruptions are felt across both the natural gas and electric systems indicating an inability to hedge against price spikes in one with the other. This price correlation also highlights the importance of crafting scenarios using data aligned within a consistent time window.
Acknowledgements
Thanks to the Heising-Simons Foundation for their generous support of this insight brief. The authors thank the following individuals for graciously offering their insights to this work. Inclusion on this list does not indicate endorsement of the findings in the insight brief.
Rebecca O’Neil, PNNL
Maria Roumpani, Current Energy Group
Mark Specht, UCS
Soh Young In, KAIST
Diego Angel Hakim, Jacob Becker, Lauren Shwisberg, Joseph Daniel, RMI
Existing utility risk assessment and mitigation processes could accommodate the addition of climate risks. The US Department of Energy and the Electric Power Research Institute (EPRI)’s Climate Resilience and Adaptation Initiative (Climate READi) lay out best practices for vulnerability assessment and resilience planning and for representing climate impacts in power system models, respectively. Exhibit 1 provides a simplified view of typical steps that show up in these and other emerging utility climate risk assessment and planning frameworks. However, we explicitly separate a step focused on distributing climate risk and overlay implicated regulatory venues, which can help findings from assessments impact utility business decisions. Finally, risk assessment processes possess a cyclical nature, with modeling assumptions, progress, and new information continuously informing the next iteration of the process.
Exhibit 1. Utility Climate Risk Planning Process
Briefly, integrating climate risk into integrated resource plans (IRPs), distribution system plans (DSPs), rate cases, and other utility planning processes comprises four main steps:
Step 1: Identify the scope of the analysis (i.e., what planning processes and modeling exercises are implicated), develop a comprehensive set of regionally relevant climate risks, collect necessary data, and determine how each risk might impact the utility business. This can occur during the resource and grid infrastructure planning processes (IRPs, DSPs, risk-specific plans such as wildfire mitigation plans) as well as inform operational decisions for existing assets.
Step 2: Assess the likelihood and severity of impacts stemming from climate risk on the utility business and customers quantitatively. This can occur during the same processes as in Step 1.
Step 3: Mitigate both exposure to and possible impacts associated with climate risks on the utility business using a portfolio of climate resilience methods. We summarize risk reduction actions in two main groups:
- Avoid exposure to climate risks where practical. For example, switching to renewable portfolios avoids exposure to fuel cost volatility.
- Reduce the impacts of climate risks where they cannot be avoided or where avoidance is cost prohibitive. For example, weatherizing power plants reduces the likelihood of frozen power plant components during winter storms.
Risk mitigation actions may occur in a variety of utility planning processes. Resource and grid infrastructure planning processes (IRPs, DSPs, risk-specific plans such as wildfire mitigation plans) offer the most direct venue for implementing climate risk mitigation measures such as generation portfolio shifts and grid hardening. Rate cases, performance-based regulation (PBR) dockets, and fuel recovery dockets also offer opportunities for risk mitigation by creating incentives for utilities to transition away from fossil fuel assets and invest in grid resilience improvement.
Step 4: Distribute the risks across different stakeholders, including ratepayers, utility investors, etc. There are two strategies for risk distribution:
- Reactive measures: After risks materialize and incur costs (e.g., fuel volatility or wildfires increase expenses), stakeholders can explore risk distribution approaches by proposing mechanisms for equitable cost allocation, particularly considering ones that alleviate the energy burden for low- and middle-income (LMI) customers and thereby preventing climate risks from producing a significant affordability crisis in disadvantaged communities. This may occur during rate cases, PBR dockets, fuel cost recovery dockets, etc.
- Proactive measures: Before the risks materialize, stakeholders can alter resource planning rules and incentive structures (e.g., fuel cost sharing) to prevent risks from materializing, balance risks among ratepayers, utilities, and investors, or transfer risks to third parties (e.g., insurance companies). This may occur in traditional resource and grid planning processes (IRPs, DSPs, risk-specific plans such as wildfire mitigation plans) or within the same regulatory venues as the reactive process (i.e., rate cases, PBR dockets, etc.).
While existing climate risk mitigation frameworks imply some distribution of risk within the risk mitigation step, we separate it here to highlight regulators’ central role in this step and point out the additional regulatory venues where risk mitigation action can take place.
This insight brief primarily focuses on Steps 1 and 2 detailing how to identify and assess climate risks. Steps 3 and 4 are discussed briefly in the later sections, with further research needs flagged for future projects.
Climate change poses financial risks to the utility business. Climate related risks fall broadly into two categories: physical risks and transition risks. The Task Force on Climate-related Financial Disclosures (TCFD) provides industry standard recommendations for disclosing climate risks falling into both categories, which have been widely adopted across companies, including some US electric utilities. The US Securities and Exchange Commission (SEC) integrated ideas from the TCFD and the GHG Protocol into their proposed climate risk disclosure requirement, which may become mandatory for public companies in the United States. Given repeated exposure to climate risks and their obligation to serve customers, disclosure is just a starting point for the utility industry.
Physical Climate Risks
Physical climate risks refer to those associated with physical impacts from climate change. Such risks may manifest as acute extreme weather events (such as historic floods, heat waves, and winter storms), or as chronic conditions that represent a new normal (such as rising average temperatures, rising sea levels, and shifting precipitation patterns). These risks can lead to more frequent reduction or disruption in the power supply, impacting reliability and resilience. Further complicating matters, some of these risks are correlated – resulting in events occurring simultaneously or in close succession that result in greater impacts.
Physical climate risks affect all customers, but the impacts of these risks differ among customers. For example, more than 25 million Americans in the Justice40 communities —which are defined as “disadvantaged communities that are marginalized by underinvestment and overburdened by pollution” – are expected to face at least 48 health-threatening heat days per year by 2050. The customers in these underserved communities, who sometimes experience affordability problems during normal days, can face increasingly difficult decisions during extreme weather events as they are driven to restrict their energy use (e.g., limit use of air conditioning during extreme heat), out of fear of unaffordable utility bills. Table A below provides an illustrative sample of the physical climate risks and possible impacts.
Table A. Descriptions of Physical Climate Risks
Transition Climate Risks
Transition climate risks are related to the impending transition to a lower carbon economy, or put differently, risks that laggards will assume by waiting or refusing to take action on the energy transition (e.g., utilities building new gas infrastructure without a plan to switch fuels or including CCS costs in planning models). Transition risks can be broken down into different categories: policy, legal, technological, market, and reputational, all of which are associated with the directive to reduce greenhouse gas emissions and transition to clean energy.
Federal policies such as the Inflation Reduction Act and Infrastructure Investment and Jobs Act have created incentives for zero-carbon technologies. On the other hand, load growth driven by datacenters and advanced manufacturing facilities top the list of drivers behind utilities’ proposals to build new gas plants, creating challenges to meet decarbonization targets set by the utility companies and state legislation. In the meantime, new or revised environmental regulations — such as the Environmental Protection Agency’s (EPA) power plant emission rules — have potentially significant impact on a utility’s operational costs. All these risks contribute to significant uncertainty in the financial impact of utility planning and investment pathways, and if not managed well, these risks may translate into unexpected bill increases. Table B below provides an illustrative sample of the transition climate risks and possible impacts.
These tables are not designed to act as an exhaustive list of all the risks that can possibly impact certain jurisdictions, and each state/utility is expected to conduct a customized assessment to identify the risks that are applicable to the local context. However, it can be a starting point for the regulators and advocates to request utilities to provide evidence around how certain risks may or may not impact their jurisdictions.
Table B. Descriptions of Transition Climate Risks
Chapter 4. Current Practices and Challenges in Climate Risk Assessments
Utilities are starting to explore practices that assess the exposure to climate risks in collaboration with industry experts. EPRI has started to consolidate resources under the Climate READi framework, and independent consultants (e.g., ICF) have started to provide climate risk assessment services to major utilities. Furthermore, some utilities are beginning to assess a subset of climate risks in IRPs, DSPs, or in dedicated risk-specific plans (e.g., wildfire mitigation plans). New York’s ConEd, Southern California Edison, Chicago’s ComEd, and Seattle City Light are leading the way with their climate plans.
Seattle City Light Climate Change Vulnerability Assessment and Adaptation Plan
In 2013, Seattle City Light’s strategic plan established objectives to research the impacts of climate change on the utility and develop an adaptation plan centered around specific mitigation actions. The plan maps eight physical climate hazards to possible impacts to five aspects of utility infrastructure resulting in thirteen total impact pathways. Finally, the report summarized adaptation actions for each aspect of utility infrastructure. While not mandated by any legislative or regulatory body, this plan benefitted from its forward thinking and the detailed description of potential actions. However, it lacks mechanisms for monitoring and enforcement.
While these efforts lay a solid foundation for establishing best practices in climate risk assessment within the planning process, utilities still face challenges in integration and data accessibility, impeding a seamless and comprehensive approach to climate-informed decision-making. For example, separate analyses are usually conducted for each utility modeling exercise (e.g., wind and solar resource forecasts, distributed energy plans, load forecasts, natural gas pipeline expansion and maintenance schedules, etc.) , and the audience and time horizon often differ among these analyses. Furthermore, access to regionally relevant high-resolution climate data and expertise to use this data correctly remains a challenge. This stems from the fact that historical weather data fails to adequately represent future climate risks and access to climate data remains uneven among utilities. Multiple organizations are actively working on solutions to address this data asymmetry – EPRI’s Climate READi and Argonne National Laboratory’s Climate Risk and Resilience (ClimRR) data portal.
Some utilities have multiple planning proceedings, with one or more dedicated to climate risk assessment, often referred to as climate resilience plans, grid security plans, etc. While this represents a solid first step, a siloed process addressing the impacts of climate change introduces additional overhead potentially increasing the difficulty of implementing findings in existing planning and operational processes.
Duke’s Carbon Plan in North Carolina
In September 2023, Duke Energy Carolinas and Duke Energy Progress released a report that provides an assessment of the climate resilience impact of its grid infrastructure investments performed by ICF. However, it focuses only on the physical risk impact of climate change (e.g., high temperature and extreme heat, extreme cold and ice, etc.) without covering transition risks, and it remains unclear what mechanism will encourage Duke to implement any of the recommendations from the analysis. Separately, Duke has been pursuing its resource planning effort through the Carbon Plan proceedings, and although their assessment of forthcoming EPA rules indicated substantial cost impacts, Duke failed to include a discussion around the impact of alternative portfolios.
Without strong regulatory guidance ahead of planning processes, utilities do not have a concrete baseline to move towards risk mitigation and allocation. When a reactive approach is taken after these risks materialize, they translate into costs for ratepayers, resulting in rising bills and stifling energy burdens for LMI customers. In the past few months, PacifiCorp filed multiple requests in several of its jurisdictions to seek deferral of the wildfire-related insurance costs and recover significant insurance premiums. These costs contribute to a portion of the request for a 26.8% rate hike for PacifiCorp’s customers in Idaho and 28.3% increase for customers in Utah, which will further elevate the energy burden in these states. Proactive measures can offer a win-win where they provide a net cost benefit or shift risk away from LMI customers.
Chapter 5. Improving Climate Risk Assessments
This section introduces additional factors that can be deployed by utilities to help better quantify climate risks and assess the distribution of climate risks across different ratepayer classes. Regulators and advocates are likely not equipped with the data and modeling resources to conduct their own analysis that deploys this framework, but they are well positioned to play a critical role in nudging the utilities to deploy or improve climate risk assessments by requesting the utilities to provide information on their analytical approaches and modeling processes. The requirements laid out by the California Public Utilities Commission can offer a starting place for other states considering climate risk assessments. The section “Questions for Regulators and Advocates on Climate Risk Planning” includes a list of targeted questions for regulators and advocates to pose in regulatory proceedings.
A climate risk assessment framework includes the following components:
- Covers the full range of physical and transition climate risks. It is critical to ensure utilities assess all the risks relevant in a utility service area rather than selecting only the ones that are in favor of their preferred portfolio. Physical climate risk identification and assessment requires expert knowledge of weather and climate science as well as how climate events can impact utility assets, whereas transition climate risk assessment requires expert knowledge of policy, economics, and finance. Weather and climate science is new territory for most utilities, so they should engage these new experts in their planning processes.
- Defines the methodology for quantifying each risk starting at the asset level and aggregating up to the portfolio level. When viewed in isolation or for an individual asset, some risks may appear manageable, but if multiple risks materialize at the same time, in quick succession, or cover a large enough part of the portfolio, the picture may change. Core to these methodologies lie two datasets:
- An hourly multi-year utility-specific weather dataset that captures a reasonable distribution of future conditions, which affect the system on operational timelines. Ideally, this dataset combines high-quality historical observational data products and high-resolution downscaled climate data. This dataset acts as the foundation for all load forecasting and production cost modeling runs.
- A 30+ year utility-specific climate dataset that captures the distributions of future temperature, precipitation, wind, and humidity to help plan for the chronic realities and extreme events of the future. This dataset acts as the foundation for long-term planning model runs, and the long-time horizon is required to capture variability across scales (e.g., extreme events, year-to-year variability, El Niño Southern Oscillation (ENSO) intensity, drought patterns, etc.). ENSO provides a key example for the necessity of a long-time horizon in climate datasets: it cycles irregularly every 2-7 years, and its intensity changes on a 10-15 year timeline. Therefore, a dataset spanning less than 7 years may only capture one aspect of the ENSO cycle and any dataset shorter than 15 years may only capture a single level of intensity. Furthermore, a 30-year dataset is hardly adequate for capturing extreme events — containing at best a 1-in-30-year event. While this meets the 1-in-10-year criteria applied in many resource adequacy studies, it fails to capture the 100-year events experienced by so many utilities in the past several years. In other words, extreme event modeling in particular requires a dataset much longer than those traditionally used in utility planning, but is necessary to adequately characterize the range of conditions that proposed 20–50 year assets will experience between today and 2075.
- Specifies exactly how and where each quantified risk feeds into utility planning models. For example, load forecasting, production cost modeling, and capacity expansion modeling for IRPs, DSPs, etc. It can also define a strategy for how the decision-making process addressing uncertainty.
Exhibit 2 below provides a conceptual illustration of how an improved climate risk assessment might affect portfolio selection in an IRP. In the scenario where no climate risk is considered (the “Base Scenario Cost” bar), capacity expansion modeling may produce a fossil-heavy portfolio that’s financially competitive compared to a clean energy portfolio. By integrating a climate risk assessment with capacity expansion and production cost modeling, planners can estimate previously externalized costs (the “Climate Risk Impact” bar), which may result in a lower overall cost for the clean energy portfolio.
Exhibit 2. Illustrative Risk Assessment Comparison
A large fraction of financial risks come from market uncertainties and fluctuating operating expenses, which are costs that are mostly classified as energy-related (i.e., variable) costs. Energy-related costs have a disproportionate impact on residential customers because, most often, the largest share of energy-related costs is allocated to the residential class in the cost allocation process. Additionally, commercial and industrial customers, who pay a larger fraction of demand-related costs through demand charges, have accessibility to hedging and direct procurement opportunities, which insulate them from the impact of high energy-related costs. In other words, clean energy portfolios – much less prone to market fluctuation due to fuel cost uncertainties and overall market uncertainties once they are in service – create more robust benefits for residential customers, including the ones that live in disadvantaged communities.
Quantitative Example of Deploying an Improved Modeling Framework
To demonstrate how this improved modeling framework works, we present an example that captures a subset of diverse climate risks and how their impacts are layered upon one another, creating a more expensive future compared to a simple baseline cost assumption. In this example, we create two sample portfolios: The gas scenario builds new gas resources, and the renewable scenario builds equal parts wind and solar with the same capacity as the gas resources. We assume that the rest of the portfolio is identical between the two scenarios, and therefore exclude it for brevity. Exhibit 3 below illustrates the ratepayer cost savings in the renewable scenario relative to the gas scenario, when both scenarios are exposed to a set of climate risks.Exhibit 3. Total Climate Risk Assessment Cost Comparison
Digging into the possible impact of each risk, CCS retrofits, carbon taxes, and natural gas prices stand out as the largest drivers of cost increase in the gas scenario, highlighting the uncertainty surrounding tightening carbon regulations and EPA rules. Carbon taxes or regulations are often qualitatively discussed or quantitatively assessed in sensitivity scenarios that do not directly inform the resource portfolio decision. Volatile natural gas prices and purchase cost increases, on the other hand, are often reflected in the IRP modeling, but utilities systematically underestimate the impact of extreme weather events on gas prices and purchase costs, leading to an insufficient picture of these risks in preferred portfolio resource modeling. We used moderate assumptions in this example, yet the gas scenario costs exceed the renewables scenario costs by 68%. In a complete implementation, each risk would ideally be represented by a probabilistic distribution. Methodological details underlying this example are provided in Appendix 1 and more detailed results are provided in Appendix 2.
Questions for Regulators and Advocates on Climate Risk Planning
The questions that we lay out in this section represent a starting point to help regulators investigate a utility’s process for identifying and assessing climate risks but are by no means exhaustive.
Table C. Discovery Questions for Regulators and Advocates
PUC Review Expectation
Discovery Questions
High-level Consideration: How is the utility accounting for physical climate risks during planning?
Utility employs an hourly multi-year utility-specific weather dataset.
- Please describe the historical weather data used in all modeling activities including the spatial and temporal resolution, the geographic coverage, and the time horizon.
- If the dataset’s temporal resolution is lower than hourly, explain what steps you’ve taken to capture the relevant intraday variability in all meteorological variables.
- If the dataset covers only a single year, explain what steps you’ve taken to account for the full range of past extreme events.
- If the temporal resolution is greater than 2km, explain what steps you’ve taken to correct for terrain effects lost at lower resolutions.
Utility employs a 30+ year utility-specific climate dataset.
- Please describe your process for downscaling climate data to produce a relevant dataset for your jurisdiction (i.e., what climate data product(s) are you using, is your process statistical or dynamical, what are the final spatial and temporal resolution, the geographic coverage, and the time horizon of the resulting dataset).
- Describe what combination of historical and projected data you use in load forecasting, capacity expansion, and projection cost modeling activities. If you are not using any projected data, explain how you’re capturing future conditions. If you are using projected data, explain the process by which you projected that data and the relationship between this data and your downscaled climate data.
Utility performs an assessment of all relevant physical climate risks.
- Provide statistics covering the frequency and severity of all relevant extreme weather events (e.g., heat waves, winter storms, flooding, hurricanes, or wildfires) over the entire horizon of your planning processes. If both the frequency and severity are not increasing over long time horizons, justify this trend using evidence from your climate dataset.
Utility load forecast is built upon the weather and climate datasets.
- Describe exactly how your weather and climate datasets impact your load forecast. Does your load forecast account for changing technology adoption trends (e.g., air conditioning, heat pumps, air purifiers, etc.) stemming from a gradually warming world? If not, show why using evidence from your climate dataset.
High-level Consideration: How is the utility accounting for transition climate risks during planning?
Utility performs an assessment of all relevant transition climate risks.
- Discuss individually which policy and regulatory, legal, technological, operational, and market risks you’re tracking. If you’re not tracking any risks in a certain category provide a justification.
Utility performs an assessment of all relevant policy and regulatory risks.
- Provide a detailed list of individual policies – at both the state and federal levels – that you’ve included in long-term planning.
- Did you model any type of carbon price? If so, provide explanations for the range of prices used in modeling. If you only included a carbon price in some scenarios, explain your reasoning.
Utility financial forecast includes spending on climate- and weather-related litigation.
- Provide a full list of past climate- and weather-related litigation claims, and report how much litigation-related spending appears in your forecast.
- If your forecast doesn’t show increased spending on climate- and weather-related litigation on a forward-looking basis, explain what mitigation efforts you’ve completed or planned to reduce such liabilities and their associated costs.
Utility performs technology cost sensitivity analyses.
- Explain your methodology for forecasting capital and operational expenditures for new units particularly the origin of your assumptions around clean technologies with rapidly decreasing prices (e.g., storage, solar, or wind) and emerging clean firm technologies with highly uncertain prices (e.g., long-duration storage, small modular reactors, or advanced geothermal).
Utility performs an assessment of the transition strategy of new or existing fossil resources.
- If an IRP includes continued operation of existing fossil assets or proposed new gas, provide a detailed description of your plan to comply with future rules limiting carbon dioxide emissions for both new and existing plants.
- If the plan involves fuel-switching to hydrogen, include plans and costs associated with delivering hydrogen to the proposed natural gas facility including the proposed source of the hydrogen.
- If the plan involves CCS, include plans and costs associated with transporting and sequestering CO2 produced by the proposed natural gas facility. These transport costs should differ based on the distance to the long-term CO2 sequestration facility.
- If the plan involves CCS, describe in detail locations and capacities of long-term CO2 sequestration facilities. If you plan to use a third-party off taker, report their current offtake capacity as a percentage of the amount you expect them to offtake from your power plants.
Utility performs an assessment of all relevant market risks.
- Explain quantitatively how you forecast natural gas prices over the planning horizon.
- If the price forecast is single-valued (i.e., not probabilistic), provide a justification for neglecting the uncertainty inherent in the price forecast.
- Would a persistently higher natural gas price result in different resource builds or accelerated retirements compared to your current/preferred portfolio?
- Explain qualitatively and quantitatively how you account for natural gas supply disruptions in your planning models.
- Explain how costs incurred during short-run (i.e., weather-driven) supply disruptions and long-run (i.e., geopolitics-driven) supply disruptions would impact cash flows and ratepayer bills.
- Explain quantitatively how you forecast wholesale electricity prices over the planning horizon.
- If the price forecast is single-valued (i.e., not probabilistic), provide a justification for neglecting the uncertainty inherent in the price forecast.
- Would persistently higher wholesale electricity prices result in different resource builds compared to your current/preferred portfolio?
Chapter 6. What’s Next: What Regulators Can Do to Address Climate Risks
The improvements discussed above can help utilities gain a better understanding of what climate risks exist, how they manifest in operations, and how to quantify potential impacts during the planning process. However, in no jurisdiction has a comprehensive framework, as outlined in this paper, been implemented. Fortunately, regulators and policymakers may have solutions at their disposal to address any potential gaps:
1. Require climate risk identification and assessment requirements to planning processes.
One of the best opportunities for a utility to identify climate risks and take steps to avoid or reduce those risks are forward-looking planning proceedings, including resource and distribution planning. Where these processes are already mandated, they typically require utilities to conduct load forecasting, commodity price forecasting, and are a platform by which the utility makes decision on resources. Each of these aspects of the planning process lends itself to climate risk analysis.
However, as discussed in Chapter 4, most utilities do not have regulatory guidance around assessing climate risks in planning or have siloed/uncoordinated processes that prevent a common understanding of priority climate risks — a necessary prerequisite for designing mitigation measures. Regulators can begin to address this issue by adding guidance to regulatory orders that identify what utilities should explicitly include in their filings to identify and assess climate risks and create clear instructions on how this assessment differs from separate resilience planning processes. In regions without appropriate publicly available climate data, such rulemaking activities will take time. Regulators can refer to Tables A and B for a starting point for a list of relevant risks, then ask utilities to assess the risks that are applicable to them, and provide justification for the ones that are not applicable. Table C in Chapter 4 provides a list of potential discovery questions with clear description of what the regulators and/or advocates should expect from the utility. In addition to dedicated sections in utility filings, regulators can create explicit expectations that utilities will face higher scrutiny if they cannot address certain risk assessment questions in their filing or response to the discovery questions. This creates an incentive for utilities to take a more proactive approach during planning to incorporate climate risks. Please refer to Chapter 5 for a complete discussion on how utilities can identify and assess climate risks. identify and assess climate risks.
2. Design new or leverage existing financial and regulatory incentives for utilities to transition away from fossil assets that present significantly increasing climate risks.
Fossil assets are associated with heightened physical and climate risks, creating major challenges for an affordability energy transition. Similarly, clean energy incentives display no clear connection to risk reduction. Financial and regulatory incentives can help drive utilities to proactively seek opportunities for clean energy investments, and regulators can take the following actions to create new incentives or make sure utilities are fully leveraging existing incentives:
- Require utilities to evaluate and leverage federal incentives to reduce costs and pass the savings benefits to customers.
Federal incentives, such as Energy Infrastructure Reinvestment (EIR) programs and tax credit community adders introduced in the Inflation Reduction Act (IRA), can create opportunities for clean energy redevelopment of legacy fossil sites, which produces net benefits for customers across the utility service territory — particularly in disadvantaged communities. These communities experience heightened vulnerability to climate change and extreme weather events on average.Fortunately, regulators can influence utility planning and investment decisions through many of the same venues. They can require utilities to include a section in their resource plans that detail how to leverage IRA incentives to benefit customers. They can also require utilities to establish a robust stakeholder engagement process to make sure all communities impacted by extreme weather events can participate in the conversations to evaluate investment decisions without over-indexing to any individual community.
- Create dedicated incentives for fuel cost risk management.
Electric utilities are authorized to get returns from capital-intensive investments and are typically authorized to pass 100 percent of fuel cost to customers. The fact that risks associated with fuel prices and price volatility are borne by customers creates a moral hazard and offers no incentive for the utility to manage those fuel costs. And since fuel prices are sensitive to climate risks (both physical and transition risks), better management of fuel costs and risk will mitigate associated climate risks.
Fuel cost recovery mechanisms can incentivize utility risk management. Some potential incentives include:
- Fuel-cost sharing: Companies bear part of the risk of fuel-cost volatility
- Fuel-cost true-up removal: The risk of fuel-price volatility is shifted back to utilities
- Fuel-risk reduction tariffs: Rate designs encourage utilities to better manage fuel costs and limit the risk to customers
- Planning and procurement: Process changes help reduce future fuel costs (e.g., all-source solicitation and procurement, fuel management plans, etc.)
- Strategies to increase access to information: Processes help inform regulator decisions about fuel costs (e.g., regular audits, enhanced prudence reviews, etc.)
- Efficiency ratio: A performance incentive mechanism rewards the utility for how efficiently it generates a megawatt-hour of power
Disallowance or the threat of disallowance can prevent utilities from recovering costs incurred due to uneconomic operation, which encourages utilities to manage fossil plants more actively.
3. Work with legislators and financial industry stakeholders to adequately and equitably distribute risks among taxpayers, shareholders, and ratepayers; particularly, explore ratepayer- or shareholder-backed self-insurance funds.
Regulators can reactively control costs associated with climate risks for LMI customers through energy affordability programs such as Percentage of Income Payment Plans (PIPPs). Still, there’s a broader need for regulators to work closely with state and federal legislators to explore alternative taxpayer funding opportunities to proactively distribute risks and costs. Similarly, regulators can collaborate with utilities as they engage their shareholders to explore innovative market mechanisms that efficiently transfer risks to the shareholders with the correct risk appetite.
Commercial insurance markets sometimes prove inefficient and overly expensive in managing the catastrophic risks introduced by low-probability, high-impact extreme events (e.g., wildfires and hurricanes). In these scenarios, utilities — and therefore ratepayers — might find it cheaper to bridge the gap by creating a fund for self-insurance. Of course, such funds generally require legislative backing or regulatory approval. California led the charge in this space by establishing the California Wildfire Fund in 2019 via AB 1054, which is designed to provide up to $21 billion in insurance protection for California’s three largest investor-owned utilities. Ratepayers and shareholders contribute equally to the fund. Other western states are beginning to follow suit. The Utah legislature recently passed SB224, which allows utilities to create a fire fund via ratepayer surcharge up to 50% of the utility’s Utah revenue requirement. This amounts to about $1 billion for Rocky Mountain Power.
Despite the benefits, self-insurance mechanisms place additional pressure on regulators to play an even more substantial role in reviewing the prudence of the process to make sure ratepayers don’t unfairly bear the burden of risks historically born by third parties in the commercial insurance market. Regulators can work with experts to carefully ensure utilities deploy a robust methodology to determine low-probability event risk — as part of a comprehensive risk assessment framework presented here — and set aside commensurate funds for self-insurance.
Chapter 7. Conclusion
A well-functioning risk mitigation strategy requires three main ingredients: an accurate and comprehensive assessment of overall risk, a responsive approach to mitigation measure design, and an equitable way of distributing the costs of both extreme event disaster response and mitigation measures. Many utility strategies currently lack one or more ingredients for climate risks. As the energy transition accelerates, and extreme weather spending along with it, regulators can take actions to ensure that utilities identify, assess, reduce, and distribute climate risks equitably — whether costs materialize retroactively or proactively. None of these steps can be implemented overnight and it may take time for the impact to be seen, but the earlier regulators and utilities take action, the more likely we can beat the speed of climate disasters while addressing reliability and affordability challenges.
Appendix 1: Methodology Overview of the Quantitative Example
Scenario data
For the quantitative example, we use wind, solar, and natural gas combined cycle (CC) data from the National Renewable Energy Laboratory (NREL) Electrification Futures Study (EFS). Specifically, we use aggregated existing natural gas numbers for capacity and generation from one EFS region for the gas scenario. We created the wind and solar scenario by splitting the capacity of the gas scenario between wind and solar while maintaining key normalized quantities (i.e., capacity factor, fixed cost per MW, and variable cost per MWh). As detailed in the EFS, future capacity factors of gas CC units are low and decrease over time. Therefore, the total generation in the wind and solar scenario is approximately 250% greater than that for the gas scenario. Since we are only using data at annual resolution all the substantial intra-annual variability in wind and solar resources are averaged out in our analysis. However, this work is focused on demonstrating the framework rather than carrying out a robust quantification of the climate risks in an individual region. When this framework is applied in practice, spatially and temporally consistent and granular data are essential.
Quantification of climate risks
In quantifying individual climate risks, we sought straightforward methods based on publicly available data or assumptions. For the impact of temperature on fuel cost we adopted the medium scenario assumption from In et al. — a 0.10% increase per year. We assume a carbon tax equal to $51/ton in 2020 dollars, which is the central estimate of the Interagency Working Group. Carbon capture and storage retrofit costs are included in the 2023 version of the NREL Annual Technology Baseline (ATB). We used ATB assumptions for estimating the total natural gas CC retrofit cost as well as increased operating costs. We further assumed that all natural gas CC units installed CCS technology to abate 90% of emissions in the year 2032. While these assumptions do account for inflation, they don’t account for the additional financing costs incurred by utilities when they build and depreciate capital assets over time, so our CCS estimate is likely conservative. For our natural gas price scenarios, we pulled daily Henry Hub spot prices and calculated annual medians for 2020-2022. We used these medians $1.90, $3.67, and $6.40 per MMBtu for the low, medium, and high fuel cost scenarios, respectively. To calculate fuel consumption from generation, we used the natural gas CC heat rate from the 2022 version of EIA-860. Finally, we used Lawrence Berkeley National Laboratory’s (LBNL) ReWEP tool to determine historical average wholesale prices for all ISOs. To remain temporally coincident with the natural gas price scenarios, we used median wholesale prices from 2020-2022 for the low, medium, and high wholesale price scenarios, which were $24.7, $46.6, and $67.8 per MWh, respectively. When inflation adjustments were performed (i.e., not inherited by the upstream data), we assumed inflation was 2.4%.
Appendix 2: Detailed Results from the Quantitative Example
Exhibit A1. Impact of higher air temperature on fuel cost
While modest, higher air temperatures reduce the efficiency of fossil plants, resulting in higher fuel consumption over time (Exhibit A1). This leads to fuel cost pass throughs to ratepayers gradually increasing over time.
Exhibit A2. Impact of carbon tax on total cost
Carbon taxes may be structured in several different ways. In any case, they increase the operating cost for fossil units (Exhibit A2), and the magnitude of the increase depends on the quantity of electricity produced by the plant as well as its marginal emissions rate. If treated similarly to other operating costs, these would be 100% rate-based, giving utilities an incentive to incur these costs rather than reduce them.
Exhibit A3. Impact of carbon capture retrofit costs on total gas unit costs
As shown in Exhibit A3, retrofitting a natural gas plant with CCS roughly equals the amount spent on OPEX over the modeling period while also increasing the OPEX marginally. Under the traditional cost-of-service regulation (COSR), utilities are encouraged to pursue such capital-intensive retrofit projects over other alternatives, which is known as the perverse incentive called capex bias. Some gas with CCS may provide an economically viable option as part of a net-zero portfolio, but utilities sometimes select gas with CCS over cleaner technologies, which likely results from outdated cost assumptions.
Exhibit A4. Impact of higher natural gas prices
Unlike retrofitting natural gas plants with CCS, volatile natural gas prices are often reflected in utility IRPs today. Since fuel costs are often passed through directly to customers, utilities have little to gain if fuel costs are higher than expected. However, underestimating natural gas price volatility could contribute to the narrative for explaining new gas over clean energy in preferred portfolios, so questioning natural gas price assumptions in IRPs remains a key role for regulators. The “Low” scenario in Figure A4, utilizes a 2020 baseline cost, showing the impact that a major event can have on natural gas demand and, in turn, prices. On the other end, the “High” scenario utilizes 2022 as the baseline, which coincides with Russia’s invasion of Ukraine. While it’s infeasible to predict such massive geopolitical or economic disruptions, the global system is exposed to a sufficient number of transition risks that utilities can expect more volatile prices in general and can modernize their approaches for forecasting price disruptions associated with extreme weather, which are expected to increase in both frequency and intensity in the coming years.
Exhibit A5. Impact of higher purchase costs
Regulated utilities purchase power from wholesale markets at vastly different fractions across the country, and purchased power is generally passed through directly to ratepayers. Naturally, a divide exists between those utilities that participate in a regional wholesale market with those that do not. However, wholesale prices are one of the best proxies available to determine how well a system as a whole is preparing for extreme weather events. Frequent extreme wholesale prices indicate poor performance and ideally incentivize additional generation to interconnect. All three scenarios shown in Exhibit A5 correspond to the same baseline years as the natural gas scenarios (i.e., 2020 for Low, 2021 for Medium, and 2022 for High), which illustrates the correlation between high natural gas prices and high wholesale purchase costs. In other words, the same geopolitical, economic, and extreme event disruptions are felt across both the natural gas and electric systems indicating an inability to hedge against price spikes in one with the other. This price correlation also highlights the importance of crafting scenarios using data aligned within a consistent time window.
Acknowledgements
Thanks to the Heising-Simons Foundation for their generous support of this insight brief. The authors thank the following individuals for graciously offering their insights to this work. Inclusion on this list does not indicate endorsement of the findings in the insight brief.
Rebecca O’Neil, PNNL
Maria Roumpani, Current Energy Group
Mark Specht, UCS
Soh Young In, KAIST
Diego Angel Hakim, Jacob Becker, Lauren Shwisberg, Joseph Daniel, RMI
Utilities are starting to explore practices that assess the exposure to climate risks in collaboration with industry experts. EPRI has started to consolidate resources under the Climate READi framework, and independent consultants (e.g., ICF) have started to provide climate risk assessment services to major utilities. Furthermore, some utilities are beginning to assess a subset of climate risks in IRPs, DSPs, or in dedicated risk-specific plans (e.g., wildfire mitigation plans). New York’s ConEd, Southern California Edison, Chicago’s ComEd, and Seattle City Light are leading the way with their climate plans.
Seattle City Light Climate Change Vulnerability Assessment and Adaptation Plan
In 2013, Seattle City Light’s strategic plan established objectives to research the impacts of climate change on the utility and develop an adaptation plan centered around specific mitigation actions. The plan maps eight physical climate hazards to possible impacts to five aspects of utility infrastructure resulting in thirteen total impact pathways. Finally, the report summarized adaptation actions for each aspect of utility infrastructure. While not mandated by any legislative or regulatory body, this plan benefitted from its forward thinking and the detailed description of potential actions. However, it lacks mechanisms for monitoring and enforcement.
While these efforts lay a solid foundation for establishing best practices in climate risk assessment within the planning process, utilities still face challenges in integration and data accessibility, impeding a seamless and comprehensive approach to climate-informed decision-making. For example, separate analyses are usually conducted for each utility modeling exercise (e.g., wind and solar resource forecasts, distributed energy plans, load forecasts, natural gas pipeline expansion and maintenance schedules, etc.) , and the audience and time horizon often differ among these analyses. Furthermore, access to regionally relevant high-resolution climate data and expertise to use this data correctly remains a challenge. This stems from the fact that historical weather data fails to adequately represent future climate risks and access to climate data remains uneven among utilities. Multiple organizations are actively working on solutions to address this data asymmetry – EPRI’s Climate READi and Argonne National Laboratory’s Climate Risk and Resilience (ClimRR) data portal.
Some utilities have multiple planning proceedings, with one or more dedicated to climate risk assessment, often referred to as climate resilience plans, grid security plans, etc. While this represents a solid first step, a siloed process addressing the impacts of climate change introduces additional overhead potentially increasing the difficulty of implementing findings in existing planning and operational processes.
In September 2023, Duke Energy Carolinas and Duke Energy Progress released a report that provides an assessment of the climate resilience impact of its grid infrastructure investments performed by ICF. However, it focuses only on the physical risk impact of climate change (e.g., high temperature and extreme heat, extreme cold and ice, etc.) without covering transition risks, and it remains unclear what mechanism will encourage Duke to implement any of the recommendations from the analysis. Separately, Duke has been pursuing its resource planning effort through the Carbon Plan proceedings, and although their assessment of forthcoming EPA rules indicated substantial cost impacts, Duke failed to include a discussion around the impact of alternative portfolios.
Without strong regulatory guidance ahead of planning processes, utilities do not have a concrete baseline to move towards risk mitigation and allocation. When a reactive approach is taken after these risks materialize, they translate into costs for ratepayers, resulting in rising bills and stifling energy burdens for LMI customers. In the past few months, PacifiCorp filed multiple requests in several of its jurisdictions to seek deferral of the wildfire-related insurance costs and recover significant insurance premiums. These costs contribute to a portion of the request for a 26.8% rate hike for PacifiCorp’s customers in Idaho and 28.3% increase for customers in Utah, which will further elevate the energy burden in these states. Proactive measures can offer a win-win where they provide a net cost benefit or shift risk away from LMI customers.
This section introduces additional factors that can be deployed by utilities to help better quantify climate risks and assess the distribution of climate risks across different ratepayer classes. Regulators and advocates are likely not equipped with the data and modeling resources to conduct their own analysis that deploys this framework, but they are well positioned to play a critical role in nudging the utilities to deploy or improve climate risk assessments by requesting the utilities to provide information on their analytical approaches and modeling processes. The requirements laid out by the California Public Utilities Commission can offer a starting place for other states considering climate risk assessments. The section “Questions for Regulators and Advocates on Climate Risk Planning” includes a list of targeted questions for regulators and advocates to pose in regulatory proceedings.
A climate risk assessment framework includes the following components:
- Covers the full range of physical and transition climate risks. It is critical to ensure utilities assess all the risks relevant in a utility service area rather than selecting only the ones that are in favor of their preferred portfolio. Physical climate risk identification and assessment requires expert knowledge of weather and climate science as well as how climate events can impact utility assets, whereas transition climate risk assessment requires expert knowledge of policy, economics, and finance. Weather and climate science is new territory for most utilities, so they should engage these new experts in their planning processes.
- Defines the methodology for quantifying each risk starting at the asset level and aggregating up to the portfolio level. When viewed in isolation or for an individual asset, some risks may appear manageable, but if multiple risks materialize at the same time, in quick succession, or cover a large enough part of the portfolio, the picture may change. Core to these methodologies lie two datasets:
- An hourly multi-year utility-specific weather dataset that captures a reasonable distribution of future conditions, which affect the system on operational timelines. Ideally, this dataset combines high-quality historical observational data products and high-resolution downscaled climate data. This dataset acts as the foundation for all load forecasting and production cost modeling runs.
- A 30+ year utility-specific climate dataset that captures the distributions of future temperature, precipitation, wind, and humidity to help plan for the chronic realities and extreme events of the future. This dataset acts as the foundation for long-term planning model runs, and the long-time horizon is required to capture variability across scales (e.g., extreme events, year-to-year variability, El Niño Southern Oscillation (ENSO) intensity, drought patterns, etc.). ENSO provides a key example for the necessity of a long-time horizon in climate datasets: it cycles irregularly every 2-7 years, and its intensity changes on a 10-15 year timeline. Therefore, a dataset spanning less than 7 years may only capture one aspect of the ENSO cycle and any dataset shorter than 15 years may only capture a single level of intensity. Furthermore, a 30-year dataset is hardly adequate for capturing extreme events — containing at best a 1-in-30-year event. While this meets the 1-in-10-year criteria applied in many resource adequacy studies, it fails to capture the 100-year events experienced by so many utilities in the past several years. In other words, extreme event modeling in particular requires a dataset much longer than those traditionally used in utility planning, but is necessary to adequately characterize the range of conditions that proposed 20–50 year assets will experience between today and 2075.
- Specifies exactly how and where each quantified risk feeds into utility planning models. For example, load forecasting, production cost modeling, and capacity expansion modeling for IRPs, DSPs, etc. It can also define a strategy for how the decision-making process addressing uncertainty.
Exhibit 2 below provides a conceptual illustration of how an improved climate risk assessment might affect portfolio selection in an IRP. In the scenario where no climate risk is considered (the “Base Scenario Cost” bar), capacity expansion modeling may produce a fossil-heavy portfolio that’s financially competitive compared to a clean energy portfolio. By integrating a climate risk assessment with capacity expansion and production cost modeling, planners can estimate previously externalized costs (the “Climate Risk Impact” bar), which may result in a lower overall cost for the clean energy portfolio.
Exhibit 2. Illustrative Risk Assessment Comparison
A large fraction of financial risks come from market uncertainties and fluctuating operating expenses, which are costs that are mostly classified as energy-related (i.e., variable) costs. Energy-related costs have a disproportionate impact on residential customers because, most often, the largest share of energy-related costs is allocated to the residential class in the cost allocation process. Additionally, commercial and industrial customers, who pay a larger fraction of demand-related costs through demand charges, have accessibility to hedging and direct procurement opportunities, which insulate them from the impact of high energy-related costs. In other words, clean energy portfolios – much less prone to market fluctuation due to fuel cost uncertainties and overall market uncertainties once they are in service – create more robust benefits for residential customers, including the ones that live in disadvantaged communities.
To demonstrate how this improved modeling framework works, we present an example that captures a subset of diverse climate risks and how their impacts are layered upon one another, creating a more expensive future compared to a simple baseline cost assumption. In this example, we create two sample portfolios: The gas scenario builds new gas resources, and the renewable scenario builds equal parts wind and solar with the same capacity as the gas resources. We assume that the rest of the portfolio is identical between the two scenarios, and therefore exclude it for brevity. Exhibit 3 below illustrates the ratepayer cost savings in the renewable scenario relative to the gas scenario, when both scenarios are exposed to a set of climate risks.Exhibit 3. Total Climate Risk Assessment Cost Comparison
Digging into the possible impact of each risk, CCS retrofits, carbon taxes, and natural gas prices stand out as the largest drivers of cost increase in the gas scenario, highlighting the uncertainty surrounding tightening carbon regulations and EPA rules. Carbon taxes or regulations are often qualitatively discussed or quantitatively assessed in sensitivity scenarios that do not directly inform the resource portfolio decision. Volatile natural gas prices and purchase cost increases, on the other hand, are often reflected in the IRP modeling, but utilities systematically underestimate the impact of extreme weather events on gas prices and purchase costs, leading to an insufficient picture of these risks in preferred portfolio resource modeling. We used moderate assumptions in this example, yet the gas scenario costs exceed the renewables scenario costs by 68%. In a complete implementation, each risk would ideally be represented by a probabilistic distribution. Methodological details underlying this example are provided in Appendix 1 and more detailed results are provided in Appendix 2.
Questions for Regulators and Advocates on Climate Risk Planning
The questions that we lay out in this section represent a starting point to help regulators investigate a utility’s process for identifying and assessing climate risks but are by no means exhaustive.
Table C. Discovery Questions for Regulators and Advocates
PUC Review Expectation | Discovery Questions |
High-level Consideration: How is the utility accounting for physical climate risks during planning? | |
Utility employs an hourly multi-year utility-specific weather dataset. |
|
Utility employs a 30+ year utility-specific climate dataset. |
|
Utility performs an assessment of all relevant physical climate risks. |
|
Utility load forecast is built upon the weather and climate datasets. |
|
High-level Consideration: How is the utility accounting for transition climate risks during planning? | |
Utility performs an assessment of all relevant transition climate risks. |
|
Utility performs an assessment of all relevant policy and regulatory risks. |
|
Utility financial forecast includes spending on climate- and weather-related litigation. |
|
Utility performs technology cost sensitivity analyses. |
|
Utility performs an assessment of the transition strategy of new or existing fossil resources. |
|
Utility performs an assessment of all relevant market risks. |
|
Chapter 6. What’s Next: What Regulators Can Do to Address Climate Risks
The improvements discussed above can help utilities gain a better understanding of what climate risks exist, how they manifest in operations, and how to quantify potential impacts during the planning process. However, in no jurisdiction has a comprehensive framework, as outlined in this paper, been implemented. Fortunately, regulators and policymakers may have solutions at their disposal to address any potential gaps:
1. Require climate risk identification and assessment requirements to planning processes.
One of the best opportunities for a utility to identify climate risks and take steps to avoid or reduce those risks are forward-looking planning proceedings, including resource and distribution planning. Where these processes are already mandated, they typically require utilities to conduct load forecasting, commodity price forecasting, and are a platform by which the utility makes decision on resources. Each of these aspects of the planning process lends itself to climate risk analysis.
However, as discussed in Chapter 4, most utilities do not have regulatory guidance around assessing climate risks in planning or have siloed/uncoordinated processes that prevent a common understanding of priority climate risks — a necessary prerequisite for designing mitigation measures. Regulators can begin to address this issue by adding guidance to regulatory orders that identify what utilities should explicitly include in their filings to identify and assess climate risks and create clear instructions on how this assessment differs from separate resilience planning processes. In regions without appropriate publicly available climate data, such rulemaking activities will take time. Regulators can refer to Tables A and B for a starting point for a list of relevant risks, then ask utilities to assess the risks that are applicable to them, and provide justification for the ones that are not applicable. Table C in Chapter 4 provides a list of potential discovery questions with clear description of what the regulators and/or advocates should expect from the utility. In addition to dedicated sections in utility filings, regulators can create explicit expectations that utilities will face higher scrutiny if they cannot address certain risk assessment questions in their filing or response to the discovery questions. This creates an incentive for utilities to take a more proactive approach during planning to incorporate climate risks. Please refer to Chapter 5 for a complete discussion on how utilities can identify and assess climate risks. identify and assess climate risks.
2. Design new or leverage existing financial and regulatory incentives for utilities to transition away from fossil assets that present significantly increasing climate risks.
Fossil assets are associated with heightened physical and climate risks, creating major challenges for an affordability energy transition. Similarly, clean energy incentives display no clear connection to risk reduction. Financial and regulatory incentives can help drive utilities to proactively seek opportunities for clean energy investments, and regulators can take the following actions to create new incentives or make sure utilities are fully leveraging existing incentives:
- Require utilities to evaluate and leverage federal incentives to reduce costs and pass the savings benefits to customers.
Federal incentives, such as Energy Infrastructure Reinvestment (EIR) programs and tax credit community adders introduced in the Inflation Reduction Act (IRA), can create opportunities for clean energy redevelopment of legacy fossil sites, which produces net benefits for customers across the utility service territory — particularly in disadvantaged communities. These communities experience heightened vulnerability to climate change and extreme weather events on average.Fortunately, regulators can influence utility planning and investment decisions through many of the same venues. They can require utilities to include a section in their resource plans that detail how to leverage IRA incentives to benefit customers. They can also require utilities to establish a robust stakeholder engagement process to make sure all communities impacted by extreme weather events can participate in the conversations to evaluate investment decisions without over-indexing to any individual community.
- Create dedicated incentives for fuel cost risk management.
Electric utilities are authorized to get returns from capital-intensive investments and are typically authorized to pass 100 percent of fuel cost to customers. The fact that risks associated with fuel prices and price volatility are borne by customers creates a moral hazard and offers no incentive for the utility to manage those fuel costs. And since fuel prices are sensitive to climate risks (both physical and transition risks), better management of fuel costs and risk will mitigate associated climate risks.
Fuel cost recovery mechanisms can incentivize utility risk management. Some potential incentives include:
- Fuel-cost sharing: Companies bear part of the risk of fuel-cost volatility
- Fuel-cost true-up removal: The risk of fuel-price volatility is shifted back to utilities
- Fuel-risk reduction tariffs: Rate designs encourage utilities to better manage fuel costs and limit the risk to customers
- Planning and procurement: Process changes help reduce future fuel costs (e.g., all-source solicitation and procurement, fuel management plans, etc.)
- Strategies to increase access to information: Processes help inform regulator decisions about fuel costs (e.g., regular audits, enhanced prudence reviews, etc.)
- Efficiency ratio: A performance incentive mechanism rewards the utility for how efficiently it generates a megawatt-hour of power
Disallowance or the threat of disallowance can prevent utilities from recovering costs incurred due to uneconomic operation, which encourages utilities to manage fossil plants more actively.
3. Work with legislators and financial industry stakeholders to adequately and equitably distribute risks among taxpayers, shareholders, and ratepayers; particularly, explore ratepayer- or shareholder-backed self-insurance funds.
Regulators can reactively control costs associated with climate risks for LMI customers through energy affordability programs such as Percentage of Income Payment Plans (PIPPs). Still, there’s a broader need for regulators to work closely with state and federal legislators to explore alternative taxpayer funding opportunities to proactively distribute risks and costs. Similarly, regulators can collaborate with utilities as they engage their shareholders to explore innovative market mechanisms that efficiently transfer risks to the shareholders with the correct risk appetite.
Commercial insurance markets sometimes prove inefficient and overly expensive in managing the catastrophic risks introduced by low-probability, high-impact extreme events (e.g., wildfires and hurricanes). In these scenarios, utilities — and therefore ratepayers — might find it cheaper to bridge the gap by creating a fund for self-insurance. Of course, such funds generally require legislative backing or regulatory approval. California led the charge in this space by establishing the California Wildfire Fund in 2019 via AB 1054, which is designed to provide up to $21 billion in insurance protection for California’s three largest investor-owned utilities. Ratepayers and shareholders contribute equally to the fund. Other western states are beginning to follow suit. The Utah legislature recently passed SB224, which allows utilities to create a fire fund via ratepayer surcharge up to 50% of the utility’s Utah revenue requirement. This amounts to about $1 billion for Rocky Mountain Power.
Despite the benefits, self-insurance mechanisms place additional pressure on regulators to play an even more substantial role in reviewing the prudence of the process to make sure ratepayers don’t unfairly bear the burden of risks historically born by third parties in the commercial insurance market. Regulators can work with experts to carefully ensure utilities deploy a robust methodology to determine low-probability event risk — as part of a comprehensive risk assessment framework presented here — and set aside commensurate funds for self-insurance.
Chapter 7. Conclusion
A well-functioning risk mitigation strategy requires three main ingredients: an accurate and comprehensive assessment of overall risk, a responsive approach to mitigation measure design, and an equitable way of distributing the costs of both extreme event disaster response and mitigation measures. Many utility strategies currently lack one or more ingredients for climate risks. As the energy transition accelerates, and extreme weather spending along with it, regulators can take actions to ensure that utilities identify, assess, reduce, and distribute climate risks equitably — whether costs materialize retroactively or proactively. None of these steps can be implemented overnight and it may take time for the impact to be seen, but the earlier regulators and utilities take action, the more likely we can beat the speed of climate disasters while addressing reliability and affordability challenges.
Appendix 1: Methodology Overview of the Quantitative Example
Scenario data
For the quantitative example, we use wind, solar, and natural gas combined cycle (CC) data from the National Renewable Energy Laboratory (NREL) Electrification Futures Study (EFS). Specifically, we use aggregated existing natural gas numbers for capacity and generation from one EFS region for the gas scenario. We created the wind and solar scenario by splitting the capacity of the gas scenario between wind and solar while maintaining key normalized quantities (i.e., capacity factor, fixed cost per MW, and variable cost per MWh). As detailed in the EFS, future capacity factors of gas CC units are low and decrease over time. Therefore, the total generation in the wind and solar scenario is approximately 250% greater than that for the gas scenario. Since we are only using data at annual resolution all the substantial intra-annual variability in wind and solar resources are averaged out in our analysis. However, this work is focused on demonstrating the framework rather than carrying out a robust quantification of the climate risks in an individual region. When this framework is applied in practice, spatially and temporally consistent and granular data are essential.
Quantification of climate risks
In quantifying individual climate risks, we sought straightforward methods based on publicly available data or assumptions. For the impact of temperature on fuel cost we adopted the medium scenario assumption from In et al. — a 0.10% increase per year. We assume a carbon tax equal to $51/ton in 2020 dollars, which is the central estimate of the Interagency Working Group. Carbon capture and storage retrofit costs are included in the 2023 version of the NREL Annual Technology Baseline (ATB). We used ATB assumptions for estimating the total natural gas CC retrofit cost as well as increased operating costs. We further assumed that all natural gas CC units installed CCS technology to abate 90% of emissions in the year 2032. While these assumptions do account for inflation, they don’t account for the additional financing costs incurred by utilities when they build and depreciate capital assets over time, so our CCS estimate is likely conservative. For our natural gas price scenarios, we pulled daily Henry Hub spot prices and calculated annual medians for 2020-2022. We used these medians $1.90, $3.67, and $6.40 per MMBtu for the low, medium, and high fuel cost scenarios, respectively. To calculate fuel consumption from generation, we used the natural gas CC heat rate from the 2022 version of EIA-860. Finally, we used Lawrence Berkeley National Laboratory’s (LBNL) ReWEP tool to determine historical average wholesale prices for all ISOs. To remain temporally coincident with the natural gas price scenarios, we used median wholesale prices from 2020-2022 for the low, medium, and high wholesale price scenarios, which were $24.7, $46.6, and $67.8 per MWh, respectively. When inflation adjustments were performed (i.e., not inherited by the upstream data), we assumed inflation was 2.4%.
Appendix 2: Detailed Results from the Quantitative Example
Exhibit A1. Impact of higher air temperature on fuel cost
While modest, higher air temperatures reduce the efficiency of fossil plants, resulting in higher fuel consumption over time (Exhibit A1). This leads to fuel cost pass throughs to ratepayers gradually increasing over time.
Exhibit A2. Impact of carbon tax on total cost
Carbon taxes may be structured in several different ways. In any case, they increase the operating cost for fossil units (Exhibit A2), and the magnitude of the increase depends on the quantity of electricity produced by the plant as well as its marginal emissions rate. If treated similarly to other operating costs, these would be 100% rate-based, giving utilities an incentive to incur these costs rather than reduce them.
Exhibit A3. Impact of carbon capture retrofit costs on total gas unit costs
As shown in Exhibit A3, retrofitting a natural gas plant with CCS roughly equals the amount spent on OPEX over the modeling period while also increasing the OPEX marginally. Under the traditional cost-of-service regulation (COSR), utilities are encouraged to pursue such capital-intensive retrofit projects over other alternatives, which is known as the perverse incentive called capex bias. Some gas with CCS may provide an economically viable option as part of a net-zero portfolio, but utilities sometimes select gas with CCS over cleaner technologies, which likely results from outdated cost assumptions.
Exhibit A4. Impact of higher natural gas prices
Unlike retrofitting natural gas plants with CCS, volatile natural gas prices are often reflected in utility IRPs today. Since fuel costs are often passed through directly to customers, utilities have little to gain if fuel costs are higher than expected. However, underestimating natural gas price volatility could contribute to the narrative for explaining new gas over clean energy in preferred portfolios, so questioning natural gas price assumptions in IRPs remains a key role for regulators. The “Low” scenario in Figure A4, utilizes a 2020 baseline cost, showing the impact that a major event can have on natural gas demand and, in turn, prices. On the other end, the “High” scenario utilizes 2022 as the baseline, which coincides with Russia’s invasion of Ukraine. While it’s infeasible to predict such massive geopolitical or economic disruptions, the global system is exposed to a sufficient number of transition risks that utilities can expect more volatile prices in general and can modernize their approaches for forecasting price disruptions associated with extreme weather, which are expected to increase in both frequency and intensity in the coming years.
Exhibit A5. Impact of higher purchase costs
Regulated utilities purchase power from wholesale markets at vastly different fractions across the country, and purchased power is generally passed through directly to ratepayers. Naturally, a divide exists between those utilities that participate in a regional wholesale market with those that do not. However, wholesale prices are one of the best proxies available to determine how well a system as a whole is preparing for extreme weather events. Frequent extreme wholesale prices indicate poor performance and ideally incentivize additional generation to interconnect. All three scenarios shown in Exhibit A5 correspond to the same baseline years as the natural gas scenarios (i.e., 2020 for Low, 2021 for Medium, and 2022 for High), which illustrates the correlation between high natural gas prices and high wholesale purchase costs. In other words, the same geopolitical, economic, and extreme event disruptions are felt across both the natural gas and electric systems indicating an inability to hedge against price spikes in one with the other. This price correlation also highlights the importance of crafting scenarios using data aligned within a consistent time window.
Acknowledgements
Thanks to the Heising-Simons Foundation for their generous support of this insight brief. The authors thank the following individuals for graciously offering their insights to this work. Inclusion on this list does not indicate endorsement of the findings in the insight brief.
Rebecca O’Neil, PNNL
Maria Roumpani, Current Energy Group
Mark Specht, UCS
Soh Young In, KAIST
Diego Angel Hakim, Jacob Becker, Lauren Shwisberg, Joseph Daniel, RMI
The improvements discussed above can help utilities gain a better understanding of what climate risks exist, how they manifest in operations, and how to quantify potential impacts during the planning process. However, in no jurisdiction has a comprehensive framework, as outlined in this paper, been implemented. Fortunately, regulators and policymakers may have solutions at their disposal to address any potential gaps:
1. Require climate risk identification and assessment requirements to planning processes.
One of the best opportunities for a utility to identify climate risks and take steps to avoid or reduce those risks are forward-looking planning proceedings, including resource and distribution planning. Where these processes are already mandated, they typically require utilities to conduct load forecasting, commodity price forecasting, and are a platform by which the utility makes decision on resources. Each of these aspects of the planning process lends itself to climate risk analysis.
However, as discussed in Chapter 4, most utilities do not have regulatory guidance around assessing climate risks in planning or have siloed/uncoordinated processes that prevent a common understanding of priority climate risks — a necessary prerequisite for designing mitigation measures. Regulators can begin to address this issue by adding guidance to regulatory orders that identify what utilities should explicitly include in their filings to identify and assess climate risks and create clear instructions on how this assessment differs from separate resilience planning processes. In regions without appropriate publicly available climate data, such rulemaking activities will take time. Regulators can refer to Tables A and B for a starting point for a list of relevant risks, then ask utilities to assess the risks that are applicable to them, and provide justification for the ones that are not applicable. Table C in Chapter 4 provides a list of potential discovery questions with clear description of what the regulators and/or advocates should expect from the utility. In addition to dedicated sections in utility filings, regulators can create explicit expectations that utilities will face higher scrutiny if they cannot address certain risk assessment questions in their filing or response to the discovery questions. This creates an incentive for utilities to take a more proactive approach during planning to incorporate climate risks. Please refer to Chapter 5 for a complete discussion on how utilities can identify and assess climate risks. identify and assess climate risks.
2. Design new or leverage existing financial and regulatory incentives for utilities to transition away from fossil assets that present significantly increasing climate risks.
Fossil assets are associated with heightened physical and climate risks, creating major challenges for an affordability energy transition. Similarly, clean energy incentives display no clear connection to risk reduction. Financial and regulatory incentives can help drive utilities to proactively seek opportunities for clean energy investments, and regulators can take the following actions to create new incentives or make sure utilities are fully leveraging existing incentives:
- Require utilities to evaluate and leverage federal incentives to reduce costs and pass the savings benefits to customers.
Federal incentives, such as Energy Infrastructure Reinvestment (EIR) programs and tax credit community adders introduced in the Inflation Reduction Act (IRA), can create opportunities for clean energy redevelopment of legacy fossil sites, which produces net benefits for customers across the utility service territory — particularly in disadvantaged communities. These communities experience heightened vulnerability to climate change and extreme weather events on average.Fortunately, regulators can influence utility planning and investment decisions through many of the same venues. They can require utilities to include a section in their resource plans that detail how to leverage IRA incentives to benefit customers. They can also require utilities to establish a robust stakeholder engagement process to make sure all communities impacted by extreme weather events can participate in the conversations to evaluate investment decisions without over-indexing to any individual community.
- Create dedicated incentives for fuel cost risk management.
Electric utilities are authorized to get returns from capital-intensive investments and are typically authorized to pass 100 percent of fuel cost to customers. The fact that risks associated with fuel prices and price volatility are borne by customers creates a moral hazard and offers no incentive for the utility to manage those fuel costs. And since fuel prices are sensitive to climate risks (both physical and transition risks), better management of fuel costs and risk will mitigate associated climate risks.
Fuel cost recovery mechanisms can incentivize utility risk management. Some potential incentives include:
- Fuel-cost sharing: Companies bear part of the risk of fuel-cost volatility
- Fuel-cost true-up removal: The risk of fuel-price volatility is shifted back to utilities
- Fuel-risk reduction tariffs: Rate designs encourage utilities to better manage fuel costs and limit the risk to customers
- Planning and procurement: Process changes help reduce future fuel costs (e.g., all-source solicitation and procurement, fuel management plans, etc.)
- Strategies to increase access to information: Processes help inform regulator decisions about fuel costs (e.g., regular audits, enhanced prudence reviews, etc.)
- Efficiency ratio: A performance incentive mechanism rewards the utility for how efficiently it generates a megawatt-hour of power
Disallowance or the threat of disallowance can prevent utilities from recovering costs incurred due to uneconomic operation, which encourages utilities to manage fossil plants more actively.
3. Work with legislators and financial industry stakeholders to adequately and equitably distribute risks among taxpayers, shareholders, and ratepayers; particularly, explore ratepayer- or shareholder-backed self-insurance funds.
Regulators can reactively control costs associated with climate risks for LMI customers through energy affordability programs such as Percentage of Income Payment Plans (PIPPs). Still, there’s a broader need for regulators to work closely with state and federal legislators to explore alternative taxpayer funding opportunities to proactively distribute risks and costs. Similarly, regulators can collaborate with utilities as they engage their shareholders to explore innovative market mechanisms that efficiently transfer risks to the shareholders with the correct risk appetite.
Commercial insurance markets sometimes prove inefficient and overly expensive in managing the catastrophic risks introduced by low-probability, high-impact extreme events (e.g., wildfires and hurricanes). In these scenarios, utilities — and therefore ratepayers — might find it cheaper to bridge the gap by creating a fund for self-insurance. Of course, such funds generally require legislative backing or regulatory approval. California led the charge in this space by establishing the California Wildfire Fund in 2019 via AB 1054, which is designed to provide up to $21 billion in insurance protection for California’s three largest investor-owned utilities. Ratepayers and shareholders contribute equally to the fund. Other western states are beginning to follow suit. The Utah legislature recently passed SB224, which allows utilities to create a fire fund via ratepayer surcharge up to 50% of the utility’s Utah revenue requirement. This amounts to about $1 billion for Rocky Mountain Power.
Despite the benefits, self-insurance mechanisms place additional pressure on regulators to play an even more substantial role in reviewing the prudence of the process to make sure ratepayers don’t unfairly bear the burden of risks historically born by third parties in the commercial insurance market. Regulators can work with experts to carefully ensure utilities deploy a robust methodology to determine low-probability event risk — as part of a comprehensive risk assessment framework presented here — and set aside commensurate funds for self-insurance.
A well-functioning risk mitigation strategy requires three main ingredients: an accurate and comprehensive assessment of overall risk, a responsive approach to mitigation measure design, and an equitable way of distributing the costs of both extreme event disaster response and mitigation measures. Many utility strategies currently lack one or more ingredients for climate risks. As the energy transition accelerates, and extreme weather spending along with it, regulators can take actions to ensure that utilities identify, assess, reduce, and distribute climate risks equitably — whether costs materialize retroactively or proactively. None of these steps can be implemented overnight and it may take time for the impact to be seen, but the earlier regulators and utilities take action, the more likely we can beat the speed of climate disasters while addressing reliability and affordability challenges.
Appendix 1: Methodology Overview of the Quantitative Example
Scenario data
For the quantitative example, we use wind, solar, and natural gas combined cycle (CC) data from the National Renewable Energy Laboratory (NREL) Electrification Futures Study (EFS). Specifically, we use aggregated existing natural gas numbers for capacity and generation from one EFS region for the gas scenario. We created the wind and solar scenario by splitting the capacity of the gas scenario between wind and solar while maintaining key normalized quantities (i.e., capacity factor, fixed cost per MW, and variable cost per MWh). As detailed in the EFS, future capacity factors of gas CC units are low and decrease over time. Therefore, the total generation in the wind and solar scenario is approximately 250% greater than that for the gas scenario. Since we are only using data at annual resolution all the substantial intra-annual variability in wind and solar resources are averaged out in our analysis. However, this work is focused on demonstrating the framework rather than carrying out a robust quantification of the climate risks in an individual region. When this framework is applied in practice, spatially and temporally consistent and granular data are essential.
Quantification of climate risks
In quantifying individual climate risks, we sought straightforward methods based on publicly available data or assumptions. For the impact of temperature on fuel cost we adopted the medium scenario assumption from In et al. — a 0.10% increase per year. We assume a carbon tax equal to $51/ton in 2020 dollars, which is the central estimate of the Interagency Working Group. Carbon capture and storage retrofit costs are included in the 2023 version of the NREL Annual Technology Baseline (ATB). We used ATB assumptions for estimating the total natural gas CC retrofit cost as well as increased operating costs. We further assumed that all natural gas CC units installed CCS technology to abate 90% of emissions in the year 2032. While these assumptions do account for inflation, they don’t account for the additional financing costs incurred by utilities when they build and depreciate capital assets over time, so our CCS estimate is likely conservative. For our natural gas price scenarios, we pulled daily Henry Hub spot prices and calculated annual medians for 2020-2022. We used these medians $1.90, $3.67, and $6.40 per MMBtu for the low, medium, and high fuel cost scenarios, respectively. To calculate fuel consumption from generation, we used the natural gas CC heat rate from the 2022 version of EIA-860. Finally, we used Lawrence Berkeley National Laboratory’s (LBNL) ReWEP tool to determine historical average wholesale prices for all ISOs. To remain temporally coincident with the natural gas price scenarios, we used median wholesale prices from 2020-2022 for the low, medium, and high wholesale price scenarios, which were $24.7, $46.6, and $67.8 per MWh, respectively. When inflation adjustments were performed (i.e., not inherited by the upstream data), we assumed inflation was 2.4%.
Appendix 2: Detailed Results from the Quantitative Example
Exhibit A1. Impact of higher air temperature on fuel cost
While modest, higher air temperatures reduce the efficiency of fossil plants, resulting in higher fuel consumption over time (Exhibit A1). This leads to fuel cost pass throughs to ratepayers gradually increasing over time.
Exhibit A2. Impact of carbon tax on total cost
Carbon taxes may be structured in several different ways. In any case, they increase the operating cost for fossil units (Exhibit A2), and the magnitude of the increase depends on the quantity of electricity produced by the plant as well as its marginal emissions rate. If treated similarly to other operating costs, these would be 100% rate-based, giving utilities an incentive to incur these costs rather than reduce them.
Exhibit A3. Impact of carbon capture retrofit costs on total gas unit costs
As shown in Exhibit A3, retrofitting a natural gas plant with CCS roughly equals the amount spent on OPEX over the modeling period while also increasing the OPEX marginally. Under the traditional cost-of-service regulation (COSR), utilities are encouraged to pursue such capital-intensive retrofit projects over other alternatives, which is known as the perverse incentive called capex bias. Some gas with CCS may provide an economically viable option as part of a net-zero portfolio, but utilities sometimes select gas with CCS over cleaner technologies, which likely results from outdated cost assumptions.
Exhibit A4. Impact of higher natural gas prices
Unlike retrofitting natural gas plants with CCS, volatile natural gas prices are often reflected in utility IRPs today. Since fuel costs are often passed through directly to customers, utilities have little to gain if fuel costs are higher than expected. However, underestimating natural gas price volatility could contribute to the narrative for explaining new gas over clean energy in preferred portfolios, so questioning natural gas price assumptions in IRPs remains a key role for regulators. The “Low” scenario in Figure A4, utilizes a 2020 baseline cost, showing the impact that a major event can have on natural gas demand and, in turn, prices. On the other end, the “High” scenario utilizes 2022 as the baseline, which coincides with Russia’s invasion of Ukraine. While it’s infeasible to predict such massive geopolitical or economic disruptions, the global system is exposed to a sufficient number of transition risks that utilities can expect more volatile prices in general and can modernize their approaches for forecasting price disruptions associated with extreme weather, which are expected to increase in both frequency and intensity in the coming years.
Exhibit A5. Impact of higher purchase costs
Regulated utilities purchase power from wholesale markets at vastly different fractions across the country, and purchased power is generally passed through directly to ratepayers. Naturally, a divide exists between those utilities that participate in a regional wholesale market with those that do not. However, wholesale prices are one of the best proxies available to determine how well a system as a whole is preparing for extreme weather events. Frequent extreme wholesale prices indicate poor performance and ideally incentivize additional generation to interconnect. All three scenarios shown in Exhibit A5 correspond to the same baseline years as the natural gas scenarios (i.e., 2020 for Low, 2021 for Medium, and 2022 for High), which illustrates the correlation between high natural gas prices and high wholesale purchase costs. In other words, the same geopolitical, economic, and extreme event disruptions are felt across both the natural gas and electric systems indicating an inability to hedge against price spikes in one with the other. This price correlation also highlights the importance of crafting scenarios using data aligned within a consistent time window.
Acknowledgements
Thanks to the Heising-Simons Foundation for their generous support of this insight brief. The authors thank the following individuals for graciously offering their insights to this work. Inclusion on this list does not indicate endorsement of the findings in the insight brief.
Rebecca O’Neil, PNNL
Maria Roumpani, Current Energy Group
Mark Specht, UCS
Soh Young In, KAIST
Diego Angel Hakim, Jacob Becker, Lauren Shwisberg, Joseph Daniel, RMI
Scenario data
For the quantitative example, we use wind, solar, and natural gas combined cycle (CC) data from the National Renewable Energy Laboratory (NREL) Electrification Futures Study (EFS). Specifically, we use aggregated existing natural gas numbers for capacity and generation from one EFS region for the gas scenario. We created the wind and solar scenario by splitting the capacity of the gas scenario between wind and solar while maintaining key normalized quantities (i.e., capacity factor, fixed cost per MW, and variable cost per MWh). As detailed in the EFS, future capacity factors of gas CC units are low and decrease over time. Therefore, the total generation in the wind and solar scenario is approximately 250% greater than that for the gas scenario. Since we are only using data at annual resolution all the substantial intra-annual variability in wind and solar resources are averaged out in our analysis. However, this work is focused on demonstrating the framework rather than carrying out a robust quantification of the climate risks in an individual region. When this framework is applied in practice, spatially and temporally consistent and granular data are essential.
Quantification of climate risks
In quantifying individual climate risks, we sought straightforward methods based on publicly available data or assumptions. For the impact of temperature on fuel cost we adopted the medium scenario assumption from In et al. — a 0.10% increase per year. We assume a carbon tax equal to $51/ton in 2020 dollars, which is the central estimate of the Interagency Working Group. Carbon capture and storage retrofit costs are included in the 2023 version of the NREL Annual Technology Baseline (ATB). We used ATB assumptions for estimating the total natural gas CC retrofit cost as well as increased operating costs. We further assumed that all natural gas CC units installed CCS technology to abate 90% of emissions in the year 2032. While these assumptions do account for inflation, they don’t account for the additional financing costs incurred by utilities when they build and depreciate capital assets over time, so our CCS estimate is likely conservative. For our natural gas price scenarios, we pulled daily Henry Hub spot prices and calculated annual medians for 2020-2022. We used these medians $1.90, $3.67, and $6.40 per MMBtu for the low, medium, and high fuel cost scenarios, respectively. To calculate fuel consumption from generation, we used the natural gas CC heat rate from the 2022 version of EIA-860. Finally, we used Lawrence Berkeley National Laboratory’s (LBNL) ReWEP tool to determine historical average wholesale prices for all ISOs. To remain temporally coincident with the natural gas price scenarios, we used median wholesale prices from 2020-2022 for the low, medium, and high wholesale price scenarios, which were $24.7, $46.6, and $67.8 per MWh, respectively. When inflation adjustments were performed (i.e., not inherited by the upstream data), we assumed inflation was 2.4%.
Exhibit A1. Impact of higher air temperature on fuel cost
While modest, higher air temperatures reduce the efficiency of fossil plants, resulting in higher fuel consumption over time (Exhibit A1). This leads to fuel cost pass throughs to ratepayers gradually increasing over time.
Exhibit A2. Impact of carbon tax on total cost
Carbon taxes may be structured in several different ways. In any case, they increase the operating cost for fossil units (Exhibit A2), and the magnitude of the increase depends on the quantity of electricity produced by the plant as well as its marginal emissions rate. If treated similarly to other operating costs, these would be 100% rate-based, giving utilities an incentive to incur these costs rather than reduce them.
Exhibit A3. Impact of carbon capture retrofit costs on total gas unit costs
As shown in Exhibit A3, retrofitting a natural gas plant with CCS roughly equals the amount spent on OPEX over the modeling period while also increasing the OPEX marginally. Under the traditional cost-of-service regulation (COSR), utilities are encouraged to pursue such capital-intensive retrofit projects over other alternatives, which is known as the perverse incentive called capex bias. Some gas with CCS may provide an economically viable option as part of a net-zero portfolio, but utilities sometimes select gas with CCS over cleaner technologies, which likely results from outdated cost assumptions.
Exhibit A4. Impact of higher natural gas prices
Unlike retrofitting natural gas plants with CCS, volatile natural gas prices are often reflected in utility IRPs today. Since fuel costs are often passed through directly to customers, utilities have little to gain if fuel costs are higher than expected. However, underestimating natural gas price volatility could contribute to the narrative for explaining new gas over clean energy in preferred portfolios, so questioning natural gas price assumptions in IRPs remains a key role for regulators. The “Low” scenario in Figure A4, utilizes a 2020 baseline cost, showing the impact that a major event can have on natural gas demand and, in turn, prices. On the other end, the “High” scenario utilizes 2022 as the baseline, which coincides with Russia’s invasion of Ukraine. While it’s infeasible to predict such massive geopolitical or economic disruptions, the global system is exposed to a sufficient number of transition risks that utilities can expect more volatile prices in general and can modernize their approaches for forecasting price disruptions associated with extreme weather, which are expected to increase in both frequency and intensity in the coming years.
Exhibit A5. Impact of higher purchase costs
Regulated utilities purchase power from wholesale markets at vastly different fractions across the country, and purchased power is generally passed through directly to ratepayers. Naturally, a divide exists between those utilities that participate in a regional wholesale market with those that do not. However, wholesale prices are one of the best proxies available to determine how well a system as a whole is preparing for extreme weather events. Frequent extreme wholesale prices indicate poor performance and ideally incentivize additional generation to interconnect. All three scenarios shown in Exhibit A5 correspond to the same baseline years as the natural gas scenarios (i.e., 2020 for Low, 2021 for Medium, and 2022 for High), which illustrates the correlation between high natural gas prices and high wholesale purchase costs. In other words, the same geopolitical, economic, and extreme event disruptions are felt across both the natural gas and electric systems indicating an inability to hedge against price spikes in one with the other. This price correlation also highlights the importance of crafting scenarios using data aligned within a consistent time window.
Acknowledgements
Thanks to the Heising-Simons Foundation for their generous support of this insight brief. The authors thank the following individuals for graciously offering their insights to this work. Inclusion on this list does not indicate endorsement of the findings in the insight brief.
Rebecca O’Neil, PNNL
Maria Roumpani, Current Energy Group
Mark Specht, UCS
Soh Young In, KAIST
Diego Angel Hakim, Jacob Becker, Lauren Shwisberg, Joseph Daniel, RMI
Thanks to the Heising-Simons Foundation for their generous support of this insight brief. The authors thank the following individuals for graciously offering their insights to this work. Inclusion on this list does not indicate endorsement of the findings in the insight brief.
Rebecca O’Neil, PNNL
Maria Roumpani, Current Energy Group
Mark Specht, UCS
Soh Young In, KAIST
Diego Angel Hakim, Jacob Becker, Lauren Shwisberg, Joseph Daniel, RMI