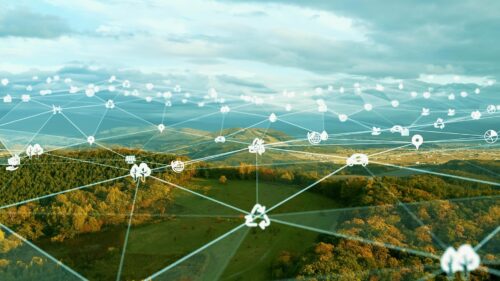
Expanding Our Vision of Energy Efficiency
Examples from buildings, transportation, and industry illustrate multilayered opportunities for greater efficiency and cost savings.
Summary
- Beginning with the end in mind: To accelerate energy efficiency, start with the overall services and apply a whole-systems approach instead of focusing exclusively on singular technology substitutions within the existing system.
- Electrification and end-use efficiency go hand in hand: Electrification promises a step change for efficiency improvements in many energy technologies. End-use efficiency facilitates electrification by reducing the size or number of energy technologies we need. Combined, both measures can reduce overall energy demand by anywhere from 50 to 75 percent.
- Energy end-use efficiency is a strong leverage point for decarbonization — and better living: Many efficiency opportunities are interconnected, spanning different sectors such as buildings, mobility, and freight transportation. For example, addressing how our cities are developed, organized, and managed is a powerful leverage point that influences all three of these sectors, plus many others, while also improving human well-being factors such as health and safety.
- Increasing efficiency awareness and mindset across stakeholders: Tapping the efficiency resource can involve many more actors than are involved in energy supply. Social tipping points, where leaders spark others to act, will be just as important as technology tipping points. Policy and regulation (including deregulation) play an especially important role to unlock solutions that are beneficial to consumers and society at large.
A new vision for energy efficiency
Deploying renewable energy and improving energy efficiency are the two primary levers for climate action between now and 2030. Agreements among nations at COP28 put these two in parallel, with the twin goals of tripling renewables and doubling the rate of efficiency improvement between now and 2030.
With renewable growth exceeding expectations, we now turn to the question: what about energy efficiency? The global agreement at COP28 calls for energy efficiency improvement rates to reach 4 percent every year until 2030. Yet when we look at global performance so far, the highest recent improvement rate is 2 percent in 2022, dipping down to 1 percent in 2023 and 2024. Reaching a 4 percent improvement rate will require a radical rethink on how we approach energy efficiency.
Traditionally, energy efficiency efforts have focused on replacing technologies, such as swapping out appliances for more efficient versions and replacing cars with higher-gas-mileage models. We offer in this insight brief a renewed and comprehensive vision for energy efficiency that includes electrification and energy end-use efficiency. Electrification promises a step change for efficiency improvements in energy technologies. Energy end-use efficiency takes a systemic approach to reducing the energy services we need while maintaining, and even improving, our quality of life. Done well, these energy efficiency measures could achieve savings and benefits that go far beyond the 4 percent target (Exhibit 1).
We analyze examples of energy- and emissions-intensive activities, starting from familiar points of reference in buildings, cars, trucking, and steel to illustrate the multilayered and interconnected nature of these energy efficiency opportunities. In each case, starting from the demand side and taking a whole-systems approach widens the scope of possible actions.
Harvesting this potential, and the many societal and environmental benefits that come with it, requires very different strategies and policies than those needed to drive renewable energy supply. Compared with supply-side approaches that focus on the largest energy buyers and sellers, delivering end-use efficiency requires a bottom-up approach to engage a diverse range of actors, including citizens, businesses, and communities, to trigger social tipping points toward greater action.
Explore two different potential paths to a 1.5°C future, with a business-as-usual outlook for comparison, in the exhibit below.
Beginning with the end in mind
Focusing on the desired end use places purposes and application before equipment, efficiency before supply, passive before active, simple before complex.
Amory Lovins, Integrative Design (2010)
Let’s take a step back and think about why we use energy in the first place. In many cases, the goal is to fulfill human needs — ranging from physiological needs such as food, hygiene, and shelter, all the way to social needs fulfilled by education, employment, and recreation. We rely on energy services to meet many of these needs. Energy services are functions performed using energy in order to achieve desired outcomes such as maintaining thermal comfort, cooking and preserving food, heating water, and getting to the places we need to be.
Energy services are provided by technologies such as cookstoves; heating, ventilation, and air conditioning (HVAC) systems; and vehicles. All these technologies generate useful energy (the energy that goes toward the desired service, e.g., heat, radiant energy, kinetic energy) using final energy (the energy a consumer purchases, e.g., metered electricity, gasoline). Final energy is provided by primary energy sources such as the sun, the wind, and fossil fuels.
Exhibit 2. Examples of Relationships between Human Well-Being, Energy Services, and Energy Technologies
Traditional energy efficiency programs often focus on specific product and appliance upgrades. These programs aim to minimize conversion losses from final energy to useful energy, such as the waste heat from a car’s combustion engine. As important as these measures are, they center on a narrow definition of energy efficiency.
When we focus on human needs, we start to see that the energy efficiency solution space extends well beyond better machines to include changing the way we fulfill human needs while generating significant energy and cost savings. Examples include walkable neighborhoods, better cycling infrastructure, passive heating and cooling, “daylighting” our buildings, and much more. These solutions are what we call energy end-use efficiency.
Energy end-use efficiency and electrification go hand in hand
Electrification offers an opportunity to radically improve efficiency in energy technologies, far beyond conventional appliance upgrades. For example, upgrading your gas furnace could reduce energy use by about 10 percent — but switching to a heat pump could reduce energy use by as much as 30–50 percent. At the same time, electrification comes with certain challenges, notably electricity load growth and affordability for users, and it only taps part of the economically available efficiency resource.
This is where energy end-use efficiency can and should play an important role. When we reduce our reliance on energy services, we also reduce the size or number of energy technologies we need. This means an easier and more affordable path to electrification: we need fewer EVs to satisfy a community’s mobility needs, and smaller — more affordable — heat pumps to satisfy people’s thermal comfort needs.
Fewer and smaller electrification technologies will also help to manage electricity demand. Again, electrification technologies are already far more efficient than fossil-based alternatives and would lessen total energy use on their own. But energy end-use efficiency takes these savings even further because the effects compound. As we show in the sector examples below, energy end-use efficiency and electrification combined can reduce overall energy demand by 50–75 percent. These savings can play a huge role in reducing burdens on the grid and easing the transition to a renewable energy future.
Attaining Thermal Comfort with Less Heating and Cooling Energy Use
Why do we use energy in buildings? One of the biggest reasons is to provide heating and cooling services. From a human perspective, the ultimate objective is to attain thermal comfort: we don’t want to be too hot, or too cold, in the buildings where we live or work.
A systems thinking approach to energy efficiency in heating and cooling entails consideration of usage, design, construction (or retrofit), and operation to identify the least-cost solutions to deliver high levels of well-being. These system measures can shrink the need for mechanical heating and cooling services and, in turn, the size and cost of heat pumps or other equipment required.
Mixed-Use, Human-Scale Development
One solution is simply to use floor space more efficiently. This is key for rapidly growing cities in the Global South, where most of the building stock is yet to be built, but it is just as relevant for growing metropolitan regions in the Global North that can achieve major impact through zoning reforms.
Multifamily homes save about 50 percent of a household’s energy compared with single-family detached homes, due to their density and shared walls, which reduce heat gain or loss. Market studies find a growing demand for compact development, especially among households without children that prioritize access and location over unit size.
Compact urban development also opens the door for district heating and cooling systems, which are most viable in high-density, mixed-use areas. District systems can reduce primary energy consumption by 30–50 percent by unlocking more efficient sources of heating and cooling — such as waste heat from data centers, large-scale heat pumps, or geothermal energy — which may be less feasible at the individual household or building level.
Passive Housing Design
There are many ways to maintain comfortable temperatures without mechanical heating or cooling. The most common example is a high-performance building envelope. In cooler climates, this means improving insulation and airtightness in the walls, roof, and foundation to minimize heat loss. Such envelope improvements also help retain cool air in warmer seasons. According to RMI’s Carbon-Free Buildings team, basic targeted weatherization, air sealing, and added insulation can typically generate energy savings of about 10–30 percent. Deep energy retrofits, which include comprehensive air sealing, added insulation, and high-performance windows and/or doors, can generate energy savings of 60–80 percent.
In hotter climates, there is an additional objective to minimize heat absorption and even emit heat. Cool roofs designed to reflect, rather than absorb, sunlight can passively reduce the internal temperature of the building and lessen the need for air conditioning. The most advanced cool roof materials don’t just reflect heat — they actively remove it from the building, lowering indoor temperatures by up to 10°C. This translates to energy savings of 20–70 percent, depending on the building type and climate.
Planting trees in urban areas can also help reduce the risk of high heat events that strain energy systems and harm human health. Trees mitigate the urban heat island effect primarily through shading and transpiration. In India, real estate developers combined various measures including building orientation, shading, and landscaping to achieve an impressive 7°C reduction in local land surface temperatures in a new community near Mumbai.
At the human level, dressing appropriately for the weather can reduce overreliance on heaters and air conditioners. Thermal variety within comfortable temperatures offers additional benefits for the human sensory experience.
Heat Pumps and Superefficient Air Conditioners
Lower demand for mechanical heating and cooling means that the same building can run on a smaller HVAC system, thereby making the investment more affordable. It also reduces the electricity demand, easing the transition to a fully renewable grid.
Heat pumps provide a leap in efficiency relative to fossil fuel heating systems and conventional compressor-based air conditioning. Because heat pumps move heat — as opposed to fossil fuel furnaces or heaters that rely on combustion to generate heat — they can produce over four times as much heat energy as they consume in electricity, saving up to half the total energy while producing zero direct emissions. Switching from fossil fuels also reduces indoor air pollution and removes potential risks of carbon monoxide poisoning and gas leaks.
Meanwhile, manufacturers have demonstrated the feasibility of superefficient air conditioners that have five times less climate impact than standard air conditioners currently available on the market.
Exhibit 3. Impacts of End-Use Efficiency Measures on Global Energy Demand from Residential Buildings in 2050
Scaling Deep Energy Retrofits in Massachusetts and California
Despite the potential of building envelope improvements, implementing retrofits often proves challenging. Deep energy retrofits require significant up-front investment, can be highly disruptive to occupants, and require owners to navigate through conflicting guidance, fragmented financing, and administrative burdens.
Inspired by innovative whole-building retrofit programs in Europe, RMI launched a market facilitation initiative for multifamily homes in Massachusetts and California to address these challenges, streamline deep energy retrofits, and demonstrate a scalable model. To make retrofits accessible for as many people as possible, particularly in historically marginalized communities, this initiative aims to facilitate a retrofit pipeline, support owners through the retrofitting process, and showcase innovative retrofit packages for common building typologies that drastically improve building performance. These efforts build market capacity and standardize the retrofit process while simultaneously aggregating demand from multifamily building owners with similar typologies.
The retrofit approach varies across building typologies and may include easily installable prefabricated insulated facade panels, insulated roof panels with solar, combined heat pump space conditioning and water heating systems, and electric appliances. Having a standardized program and common retrofit approach for each typology helps facilitate the retrofit process in several ways, including lowering information barriers for owners and shortening installation time, which reduces disruption for occupants. Standardization also makes it easier to aggregate demand and mitigate perceived risks, bringing down the price of key components.
In Massachusetts, RMI aims to have 1,000 multifamily apartment units under renovation by the end of 2024. Meanwhile, in California, RMI is demonstrating retrofit solutions on over 300,000 square feet of pilots. RMI provides further support for owners to help ease administrative and financial burdens through a streamlined application process, decarbonization assessments, assistance finding financing options, matching owners with contractors, and more.
Getting everyone to their destinations with less energy
Cars have been a primary target of energy and climate policy for many decades. Though policy success has varied, average vehicle efficiency has gradually improved, even as the number of cars on the road and the number of miles driven have continued to increase globally.
How can we radically improve energy efficiency in the mobility sector? The answer starts with land -use solutions that minimize vehicle dependence altogether, coupled with better public transit systems to enable the use of less energy-intensive transportation options such as trains and buses. Where private car usage remains necessary, electric vehicles — especially smaller, lighter ones — can offer a leap forward in efficiency.
Mixed-Use, Human-Scale Development
Strategic land use can help avoid vehicle dependence altogether by bringing key services and activities closer to one’s home. In other words, people can walk, bike, and move to places without the use of a motorized vehicle. Studies by RMI consistently find that reforms to land use policy in the United States, whether at the state or city level, can lead to per capita reductions in vehicle miles traveled (VMT) of around 10 percent.
Mixed-use and human-scale development are often attributed to urban areas but are also possible in other settings: think of the quintessential small town “Main Street” found across rural and suburban areas of the United States, or the recent burgeoning of walkable exurban downtowns. Walking and biking also offer additional health benefits, as in New York City, where bike lanes gave residents an extra year of healthy life at far lower cost than most health interventions.
Meanwhile, some private developers, like India’s Lodha Group, are choosing to develop new walkable, bikeable communities based on the market pull for this way of living. Lodha’s Palava City development, near Mumbai, follows a 5-10-15 neighborhood planning principle. This approach prioritizes having daily necessities like play areas, convenience retail, and schools within a five-minute walking distance, recreational and entertainment venues within a 10-minute walk, and necessities like healthcare facilities, banks, and offices within a 15-minute walk.
Public Transit Systems
Better transit systems can incentivize modal shifts by providing a more attractive alternative to private cars. What do “better” transit systems look like? Riders prize higher service quality in the form of increased coverage of routes and frequency of service, as well as general comfort, speed (e.g., privileged access for buses on busy roadways), safety, payment convenience, and cleanliness. Offering a wide range of options, including buses and trains but also micromobility (shared bikes and scooters), can be helpful too. Like walking and biking, better public transit also offers additional health benefits as well as equity benefits such as increased job access.
A global study by the Institute for Transportation and Development Policy (ITDP) finds that a range of measures can reduce private car activity by 50 percent, nearly halving overall energy demand from passenger transport (Exhibit 4). These measures combine transit-oriented urban development with government policies at all levels that are committed to more bicycling and pedestrian infrastructure, public transit, sustainable land use, and traffic reduction.
Electric Vehicles
Today, transformational change is afoot with the advent of competitively priced electric vehicles that are typically two to four times as efficient as cars with internal combustion engines. Because they are so efficient, EVs can reduce vehicle energy demand by 32–70 percent, depending on the source of electricity. Thus, the exponential growth of electric vehicles globally will revolutionize the efficiency of the mobility sector.
Combining electrification with the aforementioned end-use efficiency measures can bring a 67 percent reduction in energy demand from urban passenger transport, according to the same ITDP study.
Exhibit 4. Impacts of End-Use Efficiency Measures on Global Energy Demand from Urban Passenger Transport in 2050
Smaller, Lighter Cars
Basic physics dictates that heavier things require more energy to move. The weight of cars can be reduced by using lightweight materials such as high-strength steel, magnesium alloys, aluminum alloys, carbon fiber, and polymer composites. Experts estimate that vehicle weight can be lowered by 35 percent at reasonable cost, which could reduce fuel consumption by 12–20 percent with no trade-offs in terms of safety or performance. The cost of these lightweight materials can be offset at the point of manufacturing by the lower price of smaller motors and engines, even before considering fuel cost savings for the consumer.
Beyond materials, another key factor is size. Bigger cars weigh more and require more energy to move. Cars have grown steadily over the years, driven by the fact that vehicle models get bigger with every design generation, as well as by an overall market shift from compact cars and sedans to SUVs. The impact of car size is not to be underestimated: the Global Fuel Economy Initiative finds that without the shift to SUVs, more than half of vehicle weight increases could have been avoided between 2010 and 2022.
Making cars smaller and lighter will also ease the transition to electric cars. A smaller and lighter electric car needs a smaller battery, which makes it more affordable and faster to charge. Smaller and lighter cars will also improve road safety: in the event of a collision, larger, heavier cars increase the risk of severe injury or death for cyclists, pedestrians, and drivers of smaller vehicles, while offering diminishing returns in terms of safety for the driver of the larger vehicle.
Urban Transportation in Three Cities: Tokyo, Paris, and Bogotá
Tokyo is renowned for its public transportation system. The coverage of the subway system, and the frequency at which trains run, far eclipses most American cities. You’re never more than 5 to 10 minutes from a subway station, and trains run about every 5 minutes (or even less, depending on the line). On top of that, they are clean and rarely late.
Because mixed-use development is the norm in most areas of Tokyo, most people do not need cars in their daily lives. About a quarter of Tokyo residents do not have driver’s licenses. Only 50 percent own a car — down from 60 percent a decade ago — and one-third of those are compact or Kei cars. Less than 3 percent own trucks or vans. All in all, this means that transportation energy use in Tokyo is just 18 GJ per capita, compared with 47 GJ per capita in New York, while helping people get to the places they want or need to be.
It may feel challenging to transform cities at this scale, but it is possible, and we have seen it happen.
Paris began its transformation into a “100 percent bikeable city” in 2015 with the Cycling Plan (Plan Vélo). More than 70 percent of the city’s parking spaces were removed and replaced with pedestrian and cycling infrastructure. More trips are now made by bike than by car — both within the city as well as for trips between the suburbs and the city center. In the past two decades, driving within Paris has decreased by 60 percent, mirrored by a comparable increase in walking, bicycling, and public transit.
Bogotá went through a similar change two decades ago, though its experience since offers a more cautionary tale. In the early 2000s Bogotá developed the TransMilenio, a rapid bus system, to address the city’s rapidly growing population and deteriorating transportation infrastructure. By 2012, TransMilenio operated 12 lines totaling 112 kilometers, with around 1,500 buses serving 1.5 million passenger journeys each day. Initially, it was hailed as a success, reducing congestion and improving air quality, passenger safety, and accessibility. Today, however, the New York Times calls the TransMilenio an “embattled institution.” Political turnover shifted budget priorities, which led to a decline in service despite further increases to ridership: buses were not replaced as they aged, and promised route extensions were never delivered. To truly enable optimal transportation efficiency, good urban development must be an ongoing priority with sustained investment.
Fulfilling our material needs with less freight movement
We rely on a great number of material goods in our lives, and the freight industry plays a critical role in transporting these materials and goods to where they are needed. Yet at the same time, freight transportation makes up 8 percent of global greenhouse gas emissions, a majority of which comes from road vehicles such as trucks.
The way to improve trucking efficiency is by replacing inefficient trucks with more efficient ones, right? Certainly, the emergence of cost-competitive electric trucks will be a major help. But while more efficient trucks are a big part of the solution, focusing on trucks alone obscures many other opportunities for efficiency improvement in the freight transportation system, ranging from driver behavior to the overall shape of our future economy. Together, these other levers could account for a large share of the overall savings opportunity.
Material Demand Reduction
The starting point for reducing trucking emissions is not in the trucking sector, or even the larger freight sector, but rather in basic assumptions about our future economy. One possibility comes from the Low Energy Demand (LED) scenario developed by the International Institute for Applied Systems Analysis (IIASA), which provides a widely referenced benchmark for a low-energy-demand economy. LED assesses how the quantities and types of services drive supply and requirements for energy, materials, and land. Solutions include familiar measures like product lightweighting (e.g., smaller, lighter cars), fewer cars, and more efficient use of residential floorspace — as well as additional measures such as increased product longevity, sharing and service business models, reduced loss and waste, and increased material efficiency. All these measures reduce the quantity and volume of goods that must be moved, while maintaining or improving human well-being.
There are solutions across sectors, including the freight system itself. For example, Walmart’s whole-systems approach has included working with supply chain partners to lessen the volume of packaging, lighten certain cargo, improve truck loading techniques, and more.
Combined with modal shifts to rail, which we discuss below, LED shows that freight activity can be reduced to less than half of business-as-usual scenarios such as the International Energy Agency’s Stated Policies Scenario, or STEPS (Exhibit 5).
Freight Network Improvements
In parallel with material demand reduction, there are additional opportunities to reduce trucking demand through changes to the overall freight system.
Notably, shifting freight from truck to rail can lead to significant energy and cost savings, as trucks consume nearly three times more energy per ton-km as compared with rail. While this sounds straightforward, facilitating this modal shift is complex — often because today’s long-distance rail freight routes require high shipment densities to be profitable. Solutions include the integration of long-distance and short-line rail, aided by the build-out of smaller, low-cost intermodal hubs (for rail-to-truck transfer), or logistics hubs that consolidate freight volumes across multiple firms and industries. These hubs can also help improve route optimization and reduce the distance that trucks travel empty or below capacity.
Another solution is regional trucking, which can enable more direct routes and reduce miles traveled by as much as a third when combined with practices such as local warehousing and relay trucking — and can even reduce idling time.
Battery Electric Trucks and E-Cargo Bikes
By enabling shorter, simpler trucking routes, these network improvements go hand-in-hand with electrification. Electric trucks are currently better suited to shorter routes and require reliable charging availability, though this may change as battery technologies improve. Electric trucks elevate efficiency because they can be three to seven times more efficient than diesel trucks. Depending on the electricity source, this translates to a 60–80 percent reduction in emissions.
For last-mile deliveries, e-cargo bikes and electric three-wheelers provide significant energy savings, including in many emerging market economies. E-cargo bikes, for example, use 90 percent less energy per kilometer compared with an electric van, and they provide other benefits such as improved pedestrian safety and reduced congestion.
Driver Performance
Finally, where trucking cannot be reduced or avoided, we can improve efficiency by improving driver behavior. The fuel economy of trucks is greatly influenced by factors that drivers can control, such as speed, acceleration, braking, gear selection, idling, and more. Research shows that drivers can affect fuel efficiency by more than 20 percent. There are many ways to improve driver performance, including via training, incentives, and real-time driver feedback through telematic systems.
Exhibit 5. Impacts of End-Use Efficiency Measures on Global Energy Demand from Freight Trucking in 2050
Cultivating the Efficiency Mindset in the Trucking Industry The North American Council on Freight Efficiency (NACFE) is a fuel-agnostic organization focused on promoting the development and adoption of energy efficiency in the freight industry. NACFE shows how industry partners can help to increase awareness about efficiency-related cost savings, share and disseminate learnings about best practices, and create an industry-wide culture of continuous improvement.
Since 2017, in collaboration with RMI, NACFE has hosted four high-level demonstration events (NACFE Run on Less) aimed at showcasing the feasibility and impact of various trucking efficiency measures. The first two events highlighted the full savings potential from existing technologies, including for long-haul trucks and regional haul trucks. The 2021 and 2023 Run on Less events focused on battery electric trucks and truck charging depots, showcasing how fleets can maximize their benefits and scalability.
Each event has incorporated timely issues for the trucking industry, such as the growing regional haul market and the falling costs and weights of batteries. In addition, all demonstrations have involved a wide variety of trucks in terms of route, geography, vehicle type, and use case. In doing so, they have successfully called the trucking industry’s attention to effective, ready-for-market technologies, dispelling common misunderstandings and myths.
Beyond the Run on Less events, NACFE also issues confidence reports on established technologies and guidance reports on emerging technologies, as well as hosting regular trainings and workshops to help fleet owners and other relevant stakeholders make informed decisions.
Avoiding steel emissions by reducing our need for steel
Steel is one of the largest greenhouse gas emitters in heavy industry. Accordingly, new policies have been advanced in the United States, the European Union, India, and Australia to accelerate “green” steel production through direct subsidies, export credits and loans, green procurement, and penalties for emitting CO2 from traditional steelmaking. While green steel is an important way to reduce greenhouse gas emissions, there are other potent levers to reduce the emissions associated with iron and steel while respecting planetary boundaries. Starting with the end in mind, let’s consider how and why steel is used and assess a fuller range of efficiency solutions — including demand-side levers, materials efficiency, and circularity.
How does steel contribute to our well-being? The biggest uses of steel are in buildings, vehicle manufacturing, and transport infrastructure. So, how could our cities, homes, and mobility evolve to affect the demand for steel?
Reducing Steel Demand
How much steel do we need? Potentially only half of what we think.
One study concludes that global energy demand for steel production could be 60 percent lower than in a business-as-usual scenario in 2050 (Exhibit 6) when taking full advantage of demand-side approaches such as the following:
- Material efficiency focuses on providing the same material services with fewer materials. For example, smaller cars use less steel and other materials than SUVs or pickup trucks, but they can equally fulfill day-to-day needs in most cases.
- Material substitution aims to substitute away from energy-intensive materials such as steel and concrete. For example, steel can be replaced with bio-based structural materials or advanced synthetic materials.
- Material circularity reduces energy and resources by using recycled materials. For instance, producing a unit of recycled steel uses only 10–25 percent as much energy as producing virgin steel. This is primarily because virgin steel production requires an extra step of processing (iron ore reduction) that is not required when recycling scrap steel.
Separately, Mission Possible Partnership’s Steel Transition Strategy, endorsed by major global steel manufacturers, concluded that steel demand could be reduced by 41 percent in 2050 via circularity, productivity of use, and material efficiency alone.
Green Steel Production
Where primary steel production cannot be avoided, novel iron reduction and steelmaking methods can substantially reduce emissions. Best known today is hydrogen-based direct reduction, which uses green hydrogen produced from renewable energy, but there are other emerging technologies such as molten oxide electrolysis, electrowinning, and smelting via hydrogen plasma or lasers. These methods are still very energy-intensive — highlighting the importance of other efficiency measures alongside continued improvements of these methods themselves — but they can be fully powered by renewable electricity, so they offer the best path forward to reduce emissions from primary steel production.
Exhibit 6. Impacts of End-Use Efficiency Measures on Global Energy Demand from Steel Production in 2050
Industrial Clusters and Supply Chains
The development of industrial hubs and clusters offers additional system-level opportunities to reduce energy demand and emissions from steel. For example, industrial clusters could up-level energy efficiency by repurposing waste heat and materials, sharing infrastructure, and other synergies. The energy savings from such “industrial symbiosis” across steel, cement, aluminum, and paper production could be as high as 18 percent.
Industrial hubs will become critical as steel production shifts to green hydrogen. Many planned green steel factories will be sited near hydrogen hubs. For example, the Pilbara Hydrogen Hub in Australia will colocate green steel facilities with hydrogen production and export facilities. This can enable new modes of industrial symbiosis around green hydrogen, as well as create opportunities for cost and energy savings in transporting steel, as explained in the box below.
Reimagining the Steel Value ChainThe Green Iron Corridors project, led by RMI and the Green Hydrogen Catapult, envisions a radical restructuring of the steel value chain that will enable rapid growth of green steel while delivering significant cost and energy savings.
Traditionally, steel has been produced in large integrated facilities that include both the ironmaking and steelmaking process. This means that steel production is currently sited where coal and iron ore — both key inputs for traditional steelmaking — are cheap.
However, with the rise of green steel made using green hydrogen, the calculus changes. The Green Iron Corridors project proposes splitting up the ironmaking and steelmaking processes, with ironmaking sited in locations with abundant iron ore and renewable energy, such as Australia, and steelmaking in locations with existing manufacturing capabilities and strong demand markets, such as Germany.
Transforming this value chain not only facilitates the growth of green steel, but also improves energy efficiency by avoiding unnecessary transport. Rather than shipping both iron ore and hydrogen (via an ammonia carrier), importing a finished green iron product could save up to 3.1 TWh of total electricity use for a commercial-scale plant producing two million tons of steel per year (even considering the necessary reheating step to produce steel from cold iron). Producing green hydrogen in renewable-rich regions is also more infrastructure-efficient than doing so in traditional demand centers: completely replacing the EU’s nearly 100 million tons of traditional, integrated, carbon-intensive production with green production would require 70 GW less renewable capacity in Western Australia than it would in Germany.
Multiple benefits: Lower emissions, costs, and pollution
The energy efficiency opportunities described here align with a common pattern. In each case, significant savings can come from technology change — from green steel to electrification in buildings, cars, and freight. And in each example, energy end-use efficiency can bring further savings while facilitating the adoption of new technologies.
However, energy end-use efficiency is also powerful because it has cross-cutting benefits across sectors. Exhibit 7 summarizes many of the efficiency measures we’ve highlighted, emphasizing the interconnections in yellow. Urban land use and transportation policies and planning should go hand in hand, with efficiency implications for buildings, transportation, and even the steel sector and beyond — since fewer cars means less material demand for car-related infrastructure. Similarly, material efficiency, substitution, and circularity practices can reduce demand for steel while relieving freight burdens. Less freight, in turn, contributes to less traffic — further reducing demand for transportation infrastructure.
The benefits of these efficiency measures extend beyond energy savings to human well-being, as we discuss in a separate article. Fewer cars means less traffic, which means improved air quality and lower risk of traffic accidents. More walking and cycling mean better health outcomes. Passive housing design can increase household resilience to severe weather events.
In short, energy end-use efficiency measures are systemic leverage points where one intervention can lead to broader benefits for human well-being — which is why, after all, we use energy in the first place.
Exhibit 7. The Cross-Cutting Benefits of Energy End-Use Efficiency Measures

Delivering on global goals to double energy efficiency improvement rates will not only require major technology transitions in key sectors, but also better planning, design, operation, system integration, and circularity. As Amory Lovins writes, “saving energy is like eating a lobster: if you extract only the large chunks of meat from the tail and claws and throw away the rest, you will miss a comparable amount of tasty morsels tucked in crevices.”
These changes, in turn, depend on a diverse range of actors including policymakers, private companies, civil society, and consumers themselves, supported by a social norm that values efficiency and sufficiency. Establishing this kind of awareness provides an ethic of care that inspires and aligns actions at many different levels, including strengthening support for government policies and motivating corporate energy efficiency efforts.
Valuing EfficiencyThe idea of frugality may seem passé in the context of our supercharged, consumption-oriented global economy, but some leaders today are invoking this value as a key part of the path to greater well-being. Researchers working on energy and climate have chosen the more analytical term high-with-low to describe pathways to sustainability that center demand-side solutions for high well-being with low resource demand. These pathways achieve high levels of human development while keeping human resource footprints within planetary boundaries. Research shows that while demand-side solutions are generally underemphasized in the analysis of energy transition pathways, high-with-low scenarios show great promise for delivering a sustainable future while mitigating the worst consequences of climate change.
Is it possible to revive efficiency as a social norm? Values such as efficiency and frugality have deep and cross-cultural roots. Confucius promoted moderation as central to leading a virtuous life; he believed that practicing frugality was a way to cultivate moral character, humility, and responsibility. Mohandas Gandhi said, “The world has enough for everyone’s need, but not enough for everyone’s greed” and encouraged all people to “live simply so that others may simply live.” In the early history of the United States, Benjamin Franklin coined the phrase “a penny saved is a penny earned,” and he made the ethic of frugality a constant theme of his writings in Poor Richard’s Almanac, published to the 13 colonies for over 25 years from 1732 to 1758. “Without industry and frugality nothing will do; with them, everything,” Franklin wrote.
Given the diversity of actors involved in delivering energy efficiency, refreshing the societal values of efficiency and frugality can be an important force to reinforce these actions. Is a social tipping point in this direction possible?
Here are some examples of existing actions to highlight the energy efficiency resource — and the benefits of unlocking it:
- Global: Leaders of 197 nations plus the European Union signed a Framework Convention at COP28 setting a goal to double the rate of energy efficiency improvement between the time of the agreement and 2030. The agreement raised the profile of energy efficiency among national governments, energy and climate experts, and the media. Other global efforts have aligned with this goal, including Mission Efficiency — an alliance of partners from civil society and public- and private-sector institutions urging world leaders to unlock the energy efficiency investment that is crucial to meeting climate targets.
- National: Many countries have adopted national goals and/or launched campaigns to promote energy efficiency at a national or multinational level. These include India’s Lifestyles for Environment (LiFE), a mass movement championed by Prime Minister Modi to nudge individual and community action to protect and preserve the environment. “What is needed today is mindful and deliberate utilization, instead of mindless and destructive consumption,” says Modi. Today, over 1 million schools in India are connected to eco-clubs aimed at raising awareness about sustainable lifestyles. Other awareness campaigns include the EU’s NUDGE project, which implements and evaluates different behavioral interventions for energy efficiency, paving the way for new policies and increased consumer awareness, and Japan’s “Cool Biz” campaign that encourages weather-appropriate attire for office workers. Other countries such as New Zealand and Bhutan take a more holistic approach to encourage an energy efficient — or sufficient — lifestyle by encouraging a focus on well-being.
- City and community: Many cities and communities around the world have emphasized energy efficiency in their planning, policies, and outreach. One example of a community with an integrated approach to energy and resource efficiency is Palava City, which was mentioned above as an example of walkable urban design, with parallel efforts in energy, materials, and water efficiency. The city is designed to be a “living laboratory” for continuously refining and improving sustainability practices. Another example is the Vauban district of Freiburg, Germany, where every building has rooftop solar and many are built according to passive design principles. The city is built around walking, biking, and a tramway, and it has a sustainable waste management system.
- Corporate and industry: Businesses have established numerous coalitions to increase awareness of energy efficiency opportunities and accelerate implementation of solutions. For example, EP100 is a global corporate energy efficiency initiative, led by Climate Group, bringing together more than 125 ambitious businesses committed to improving their energy efficiency. EP100 members are committed to doubling their energy productivity, rolling out energy management systems, or achieving net-zero carbon buildings. By making public commitments, and driving toward those targets, companies can show climate leadership and send a powerful signal to policymakers and other businesses about the enormous potential of energy efficiency.
Acknowledgments
Special thanks to Ari Kahn, Kaitlyn Ramirez, Mike Roeth, Eva Rosenbloom, Lucas Toffoli, Brett Webster, Rachel Wilmoth, and Nick Yavorsky for their contributions.
The authors acknowledge support from the Energy Demand changes Induced by Technological and Social innovations (EDITS) project, which is part of the initiative coordinated by the Research Institute of Innovative Technology for the Earth (RITE) and the International Institute for Applied Systems Analysis (IIASA), funded by the Ministry of Economy, Trade, and Industry (METI), Japan.
- Beginning with the end in mind: To accelerate energy efficiency, start with the overall services and apply a whole-systems approach instead of focusing exclusively on singular technology substitutions within the existing system.
- Electrification and end-use efficiency go hand in hand: Electrification promises a step change for efficiency improvements in many energy technologies. End-use efficiency facilitates electrification by reducing the size or number of energy technologies we need. Combined, both measures can reduce overall energy demand by anywhere from 50 to 75 percent.
- Energy end-use efficiency is a strong leverage point for decarbonization — and better living: Many efficiency opportunities are interconnected, spanning different sectors such as buildings, mobility, and freight transportation. For example, addressing how our cities are developed, organized, and managed is a powerful leverage point that influences all three of these sectors, plus many others, while also improving human well-being factors such as health and safety.
- Increasing efficiency awareness and mindset across stakeholders: Tapping the efficiency resource can involve many more actors than are involved in energy supply. Social tipping points, where leaders spark others to act, will be just as important as technology tipping points. Policy and regulation (including deregulation) play an especially important role to unlock solutions that are beneficial to consumers and society at large.
Deploying renewable energy and improving energy efficiency are the two primary levers for climate action between now and 2030. Agreements among nations at COP28 put these two in parallel, with the twin goals of tripling renewables and doubling the rate of efficiency improvement between now and 2030.
With renewable growth exceeding expectations, we now turn to the question: what about energy efficiency? The global agreement at COP28 calls for energy efficiency improvement rates to reach 4 percent every year until 2030. Yet when we look at global performance so far, the highest recent improvement rate is 2 percent in 2022, dipping down to 1 percent in 2023 and 2024. Reaching a 4 percent improvement rate will require a radical rethink on how we approach energy efficiency.
Traditionally, energy efficiency efforts have focused on replacing technologies, such as swapping out appliances for more efficient versions and replacing cars with higher-gas-mileage models. We offer in this insight brief a renewed and comprehensive vision for energy efficiency that includes electrification and energy end-use efficiency. Electrification promises a step change for efficiency improvements in energy technologies. Energy end-use efficiency takes a systemic approach to reducing the energy services we need while maintaining, and even improving, our quality of life. Done well, these energy efficiency measures could achieve savings and benefits that go far beyond the 4 percent target (Exhibit 1).
We analyze examples of energy- and emissions-intensive activities, starting from familiar points of reference in buildings, cars, trucking, and steel to illustrate the multilayered and interconnected nature of these energy efficiency opportunities. In each case, starting from the demand side and taking a whole-systems approach widens the scope of possible actions.
Harvesting this potential, and the many societal and environmental benefits that come with it, requires very different strategies and policies than those needed to drive renewable energy supply. Compared with supply-side approaches that focus on the largest energy buyers and sellers, delivering end-use efficiency requires a bottom-up approach to engage a diverse range of actors, including citizens, businesses, and communities, to trigger social tipping points toward greater action.
Explore two different potential paths to a 1.5°C future, with a business-as-usual outlook for comparison, in the exhibit below.
Beginning with the end in mind
Focusing on the desired end use places purposes and application before equipment, efficiency before supply, passive before active, simple before complex.
Amory Lovins, Integrative Design (2010)
Let’s take a step back and think about why we use energy in the first place. In many cases, the goal is to fulfill human needs — ranging from physiological needs such as food, hygiene, and shelter, all the way to social needs fulfilled by education, employment, and recreation. We rely on energy services to meet many of these needs. Energy services are functions performed using energy in order to achieve desired outcomes such as maintaining thermal comfort, cooking and preserving food, heating water, and getting to the places we need to be.
Energy services are provided by technologies such as cookstoves; heating, ventilation, and air conditioning (HVAC) systems; and vehicles. All these technologies generate useful energy (the energy that goes toward the desired service, e.g., heat, radiant energy, kinetic energy) using final energy (the energy a consumer purchases, e.g., metered electricity, gasoline). Final energy is provided by primary energy sources such as the sun, the wind, and fossil fuels.
Exhibit 2. Examples of Relationships between Human Well-Being, Energy Services, and Energy Technologies
Traditional energy efficiency programs often focus on specific product and appliance upgrades. These programs aim to minimize conversion losses from final energy to useful energy, such as the waste heat from a car’s combustion engine. As important as these measures are, they center on a narrow definition of energy efficiency.
When we focus on human needs, we start to see that the energy efficiency solution space extends well beyond better machines to include changing the way we fulfill human needs while generating significant energy and cost savings. Examples include walkable neighborhoods, better cycling infrastructure, passive heating and cooling, “daylighting” our buildings, and much more. These solutions are what we call energy end-use efficiency.
Energy end-use efficiency and electrification go hand in hand
Electrification offers an opportunity to radically improve efficiency in energy technologies, far beyond conventional appliance upgrades. For example, upgrading your gas furnace could reduce energy use by about 10 percent — but switching to a heat pump could reduce energy use by as much as 30–50 percent. At the same time, electrification comes with certain challenges, notably electricity load growth and affordability for users, and it only taps part of the economically available efficiency resource.
This is where energy end-use efficiency can and should play an important role. When we reduce our reliance on energy services, we also reduce the size or number of energy technologies we need. This means an easier and more affordable path to electrification: we need fewer EVs to satisfy a community’s mobility needs, and smaller — more affordable — heat pumps to satisfy people’s thermal comfort needs.
Fewer and smaller electrification technologies will also help to manage electricity demand. Again, electrification technologies are already far more efficient than fossil-based alternatives and would lessen total energy use on their own. But energy end-use efficiency takes these savings even further because the effects compound. As we show in the sector examples below, energy end-use efficiency and electrification combined can reduce overall energy demand by 50–75 percent. These savings can play a huge role in reducing burdens on the grid and easing the transition to a renewable energy future.
Attaining Thermal Comfort with Less Heating and Cooling Energy Use
Why do we use energy in buildings? One of the biggest reasons is to provide heating and cooling services. From a human perspective, the ultimate objective is to attain thermal comfort: we don’t want to be too hot, or too cold, in the buildings where we live or work.
A systems thinking approach to energy efficiency in heating and cooling entails consideration of usage, design, construction (or retrofit), and operation to identify the least-cost solutions to deliver high levels of well-being. These system measures can shrink the need for mechanical heating and cooling services and, in turn, the size and cost of heat pumps or other equipment required.
Mixed-Use, Human-Scale Development
One solution is simply to use floor space more efficiently. This is key for rapidly growing cities in the Global South, where most of the building stock is yet to be built, but it is just as relevant for growing metropolitan regions in the Global North that can achieve major impact through zoning reforms.
Multifamily homes save about 50 percent of a household’s energy compared with single-family detached homes, due to their density and shared walls, which reduce heat gain or loss. Market studies find a growing demand for compact development, especially among households without children that prioritize access and location over unit size.
Compact urban development also opens the door for district heating and cooling systems, which are most viable in high-density, mixed-use areas. District systems can reduce primary energy consumption by 30–50 percent by unlocking more efficient sources of heating and cooling — such as waste heat from data centers, large-scale heat pumps, or geothermal energy — which may be less feasible at the individual household or building level.
Passive Housing Design
There are many ways to maintain comfortable temperatures without mechanical heating or cooling. The most common example is a high-performance building envelope. In cooler climates, this means improving insulation and airtightness in the walls, roof, and foundation to minimize heat loss. Such envelope improvements also help retain cool air in warmer seasons. According to RMI’s Carbon-Free Buildings team, basic targeted weatherization, air sealing, and added insulation can typically generate energy savings of about 10–30 percent. Deep energy retrofits, which include comprehensive air sealing, added insulation, and high-performance windows and/or doors, can generate energy savings of 60–80 percent.
In hotter climates, there is an additional objective to minimize heat absorption and even emit heat. Cool roofs designed to reflect, rather than absorb, sunlight can passively reduce the internal temperature of the building and lessen the need for air conditioning. The most advanced cool roof materials don’t just reflect heat — they actively remove it from the building, lowering indoor temperatures by up to 10°C. This translates to energy savings of 20–70 percent, depending on the building type and climate.
Planting trees in urban areas can also help reduce the risk of high heat events that strain energy systems and harm human health. Trees mitigate the urban heat island effect primarily through shading and transpiration. In India, real estate developers combined various measures including building orientation, shading, and landscaping to achieve an impressive 7°C reduction in local land surface temperatures in a new community near Mumbai.
At the human level, dressing appropriately for the weather can reduce overreliance on heaters and air conditioners. Thermal variety within comfortable temperatures offers additional benefits for the human sensory experience.
Heat Pumps and Superefficient Air Conditioners
Lower demand for mechanical heating and cooling means that the same building can run on a smaller HVAC system, thereby making the investment more affordable. It also reduces the electricity demand, easing the transition to a fully renewable grid.
Heat pumps provide a leap in efficiency relative to fossil fuel heating systems and conventional compressor-based air conditioning. Because heat pumps move heat — as opposed to fossil fuel furnaces or heaters that rely on combustion to generate heat — they can produce over four times as much heat energy as they consume in electricity, saving up to half the total energy while producing zero direct emissions. Switching from fossil fuels also reduces indoor air pollution and removes potential risks of carbon monoxide poisoning and gas leaks.
Meanwhile, manufacturers have demonstrated the feasibility of superefficient air conditioners that have five times less climate impact than standard air conditioners currently available on the market.
Exhibit 3. Impacts of End-Use Efficiency Measures on Global Energy Demand from Residential Buildings in 2050
Scaling Deep Energy Retrofits in Massachusetts and California
Despite the potential of building envelope improvements, implementing retrofits often proves challenging. Deep energy retrofits require significant up-front investment, can be highly disruptive to occupants, and require owners to navigate through conflicting guidance, fragmented financing, and administrative burdens.
Inspired by innovative whole-building retrofit programs in Europe, RMI launched a market facilitation initiative for multifamily homes in Massachusetts and California to address these challenges, streamline deep energy retrofits, and demonstrate a scalable model. To make retrofits accessible for as many people as possible, particularly in historically marginalized communities, this initiative aims to facilitate a retrofit pipeline, support owners through the retrofitting process, and showcase innovative retrofit packages for common building typologies that drastically improve building performance. These efforts build market capacity and standardize the retrofit process while simultaneously aggregating demand from multifamily building owners with similar typologies.
The retrofit approach varies across building typologies and may include easily installable prefabricated insulated facade panels, insulated roof panels with solar, combined heat pump space conditioning and water heating systems, and electric appliances. Having a standardized program and common retrofit approach for each typology helps facilitate the retrofit process in several ways, including lowering information barriers for owners and shortening installation time, which reduces disruption for occupants. Standardization also makes it easier to aggregate demand and mitigate perceived risks, bringing down the price of key components.
In Massachusetts, RMI aims to have 1,000 multifamily apartment units under renovation by the end of 2024. Meanwhile, in California, RMI is demonstrating retrofit solutions on over 300,000 square feet of pilots. RMI provides further support for owners to help ease administrative and financial burdens through a streamlined application process, decarbonization assessments, assistance finding financing options, matching owners with contractors, and more.
Getting everyone to their destinations with less energy
Cars have been a primary target of energy and climate policy for many decades. Though policy success has varied, average vehicle efficiency has gradually improved, even as the number of cars on the road and the number of miles driven have continued to increase globally.
How can we radically improve energy efficiency in the mobility sector? The answer starts with land -use solutions that minimize vehicle dependence altogether, coupled with better public transit systems to enable the use of less energy-intensive transportation options such as trains and buses. Where private car usage remains necessary, electric vehicles — especially smaller, lighter ones — can offer a leap forward in efficiency.
Mixed-Use, Human-Scale Development
Strategic land use can help avoid vehicle dependence altogether by bringing key services and activities closer to one’s home. In other words, people can walk, bike, and move to places without the use of a motorized vehicle. Studies by RMI consistently find that reforms to land use policy in the United States, whether at the state or city level, can lead to per capita reductions in vehicle miles traveled (VMT) of around 10 percent.
Mixed-use and human-scale development are often attributed to urban areas but are also possible in other settings: think of the quintessential small town “Main Street” found across rural and suburban areas of the United States, or the recent burgeoning of walkable exurban downtowns. Walking and biking also offer additional health benefits, as in New York City, where bike lanes gave residents an extra year of healthy life at far lower cost than most health interventions.
Meanwhile, some private developers, like India’s Lodha Group, are choosing to develop new walkable, bikeable communities based on the market pull for this way of living. Lodha’s Palava City development, near Mumbai, follows a 5-10-15 neighborhood planning principle. This approach prioritizes having daily necessities like play areas, convenience retail, and schools within a five-minute walking distance, recreational and entertainment venues within a 10-minute walk, and necessities like healthcare facilities, banks, and offices within a 15-minute walk.
Public Transit Systems
Better transit systems can incentivize modal shifts by providing a more attractive alternative to private cars. What do “better” transit systems look like? Riders prize higher service quality in the form of increased coverage of routes and frequency of service, as well as general comfort, speed (e.g., privileged access for buses on busy roadways), safety, payment convenience, and cleanliness. Offering a wide range of options, including buses and trains but also micromobility (shared bikes and scooters), can be helpful too. Like walking and biking, better public transit also offers additional health benefits as well as equity benefits such as increased job access.
A global study by the Institute for Transportation and Development Policy (ITDP) finds that a range of measures can reduce private car activity by 50 percent, nearly halving overall energy demand from passenger transport (Exhibit 4). These measures combine transit-oriented urban development with government policies at all levels that are committed to more bicycling and pedestrian infrastructure, public transit, sustainable land use, and traffic reduction.
Electric Vehicles
Today, transformational change is afoot with the advent of competitively priced electric vehicles that are typically two to four times as efficient as cars with internal combustion engines. Because they are so efficient, EVs can reduce vehicle energy demand by 32–70 percent, depending on the source of electricity. Thus, the exponential growth of electric vehicles globally will revolutionize the efficiency of the mobility sector.
Combining electrification with the aforementioned end-use efficiency measures can bring a 67 percent reduction in energy demand from urban passenger transport, according to the same ITDP study.
Exhibit 4. Impacts of End-Use Efficiency Measures on Global Energy Demand from Urban Passenger Transport in 2050
Smaller, Lighter Cars
Basic physics dictates that heavier things require more energy to move. The weight of cars can be reduced by using lightweight materials such as high-strength steel, magnesium alloys, aluminum alloys, carbon fiber, and polymer composites. Experts estimate that vehicle weight can be lowered by 35 percent at reasonable cost, which could reduce fuel consumption by 12–20 percent with no trade-offs in terms of safety or performance. The cost of these lightweight materials can be offset at the point of manufacturing by the lower price of smaller motors and engines, even before considering fuel cost savings for the consumer.
Beyond materials, another key factor is size. Bigger cars weigh more and require more energy to move. Cars have grown steadily over the years, driven by the fact that vehicle models get bigger with every design generation, as well as by an overall market shift from compact cars and sedans to SUVs. The impact of car size is not to be underestimated: the Global Fuel Economy Initiative finds that without the shift to SUVs, more than half of vehicle weight increases could have been avoided between 2010 and 2022.
Making cars smaller and lighter will also ease the transition to electric cars. A smaller and lighter electric car needs a smaller battery, which makes it more affordable and faster to charge. Smaller and lighter cars will also improve road safety: in the event of a collision, larger, heavier cars increase the risk of severe injury or death for cyclists, pedestrians, and drivers of smaller vehicles, while offering diminishing returns in terms of safety for the driver of the larger vehicle.
Urban Transportation in Three Cities: Tokyo, Paris, and Bogotá
Tokyo is renowned for its public transportation system. The coverage of the subway system, and the frequency at which trains run, far eclipses most American cities. You’re never more than 5 to 10 minutes from a subway station, and trains run about every 5 minutes (or even less, depending on the line). On top of that, they are clean and rarely late.
Because mixed-use development is the norm in most areas of Tokyo, most people do not need cars in their daily lives. About a quarter of Tokyo residents do not have driver’s licenses. Only 50 percent own a car — down from 60 percent a decade ago — and one-third of those are compact or Kei cars. Less than 3 percent own trucks or vans. All in all, this means that transportation energy use in Tokyo is just 18 GJ per capita, compared with 47 GJ per capita in New York, while helping people get to the places they want or need to be.
It may feel challenging to transform cities at this scale, but it is possible, and we have seen it happen.
Paris began its transformation into a “100 percent bikeable city” in 2015 with the Cycling Plan (Plan Vélo). More than 70 percent of the city’s parking spaces were removed and replaced with pedestrian and cycling infrastructure. More trips are now made by bike than by car — both within the city as well as for trips between the suburbs and the city center. In the past two decades, driving within Paris has decreased by 60 percent, mirrored by a comparable increase in walking, bicycling, and public transit.
Bogotá went through a similar change two decades ago, though its experience since offers a more cautionary tale. In the early 2000s Bogotá developed the TransMilenio, a rapid bus system, to address the city’s rapidly growing population and deteriorating transportation infrastructure. By 2012, TransMilenio operated 12 lines totaling 112 kilometers, with around 1,500 buses serving 1.5 million passenger journeys each day. Initially, it was hailed as a success, reducing congestion and improving air quality, passenger safety, and accessibility. Today, however, the New York Times calls the TransMilenio an “embattled institution.” Political turnover shifted budget priorities, which led to a decline in service despite further increases to ridership: buses were not replaced as they aged, and promised route extensions were never delivered. To truly enable optimal transportation efficiency, good urban development must be an ongoing priority with sustained investment.
Fulfilling our material needs with less freight movement
We rely on a great number of material goods in our lives, and the freight industry plays a critical role in transporting these materials and goods to where they are needed. Yet at the same time, freight transportation makes up 8 percent of global greenhouse gas emissions, a majority of which comes from road vehicles such as trucks.
The way to improve trucking efficiency is by replacing inefficient trucks with more efficient ones, right? Certainly, the emergence of cost-competitive electric trucks will be a major help. But while more efficient trucks are a big part of the solution, focusing on trucks alone obscures many other opportunities for efficiency improvement in the freight transportation system, ranging from driver behavior to the overall shape of our future economy. Together, these other levers could account for a large share of the overall savings opportunity.
Material Demand Reduction
The starting point for reducing trucking emissions is not in the trucking sector, or even the larger freight sector, but rather in basic assumptions about our future economy. One possibility comes from the Low Energy Demand (LED) scenario developed by the International Institute for Applied Systems Analysis (IIASA), which provides a widely referenced benchmark for a low-energy-demand economy. LED assesses how the quantities and types of services drive supply and requirements for energy, materials, and land. Solutions include familiar measures like product lightweighting (e.g., smaller, lighter cars), fewer cars, and more efficient use of residential floorspace — as well as additional measures such as increased product longevity, sharing and service business models, reduced loss and waste, and increased material efficiency. All these measures reduce the quantity and volume of goods that must be moved, while maintaining or improving human well-being.
There are solutions across sectors, including the freight system itself. For example, Walmart’s whole-systems approach has included working with supply chain partners to lessen the volume of packaging, lighten certain cargo, improve truck loading techniques, and more.
Combined with modal shifts to rail, which we discuss below, LED shows that freight activity can be reduced to less than half of business-as-usual scenarios such as the International Energy Agency’s Stated Policies Scenario, or STEPS (Exhibit 5).
Freight Network Improvements
In parallel with material demand reduction, there are additional opportunities to reduce trucking demand through changes to the overall freight system.
Notably, shifting freight from truck to rail can lead to significant energy and cost savings, as trucks consume nearly three times more energy per ton-km as compared with rail. While this sounds straightforward, facilitating this modal shift is complex — often because today’s long-distance rail freight routes require high shipment densities to be profitable. Solutions include the integration of long-distance and short-line rail, aided by the build-out of smaller, low-cost intermodal hubs (for rail-to-truck transfer), or logistics hubs that consolidate freight volumes across multiple firms and industries. These hubs can also help improve route optimization and reduce the distance that trucks travel empty or below capacity.
Another solution is regional trucking, which can enable more direct routes and reduce miles traveled by as much as a third when combined with practices such as local warehousing and relay trucking — and can even reduce idling time.
Battery Electric Trucks and E-Cargo Bikes
By enabling shorter, simpler trucking routes, these network improvements go hand-in-hand with electrification. Electric trucks are currently better suited to shorter routes and require reliable charging availability, though this may change as battery technologies improve. Electric trucks elevate efficiency because they can be three to seven times more efficient than diesel trucks. Depending on the electricity source, this translates to a 60–80 percent reduction in emissions.
For last-mile deliveries, e-cargo bikes and electric three-wheelers provide significant energy savings, including in many emerging market economies. E-cargo bikes, for example, use 90 percent less energy per kilometer compared with an electric van, and they provide other benefits such as improved pedestrian safety and reduced congestion.
Driver Performance
Finally, where trucking cannot be reduced or avoided, we can improve efficiency by improving driver behavior. The fuel economy of trucks is greatly influenced by factors that drivers can control, such as speed, acceleration, braking, gear selection, idling, and more. Research shows that drivers can affect fuel efficiency by more than 20 percent. There are many ways to improve driver performance, including via training, incentives, and real-time driver feedback through telematic systems.
Exhibit 5. Impacts of End-Use Efficiency Measures on Global Energy Demand from Freight Trucking in 2050
Cultivating the Efficiency Mindset in the Trucking Industry The North American Council on Freight Efficiency (NACFE) is a fuel-agnostic organization focused on promoting the development and adoption of energy efficiency in the freight industry. NACFE shows how industry partners can help to increase awareness about efficiency-related cost savings, share and disseminate learnings about best practices, and create an industry-wide culture of continuous improvement.
Since 2017, in collaboration with RMI, NACFE has hosted four high-level demonstration events (NACFE Run on Less) aimed at showcasing the feasibility and impact of various trucking efficiency measures. The first two events highlighted the full savings potential from existing technologies, including for long-haul trucks and regional haul trucks. The 2021 and 2023 Run on Less events focused on battery electric trucks and truck charging depots, showcasing how fleets can maximize their benefits and scalability.
Each event has incorporated timely issues for the trucking industry, such as the growing regional haul market and the falling costs and weights of batteries. In addition, all demonstrations have involved a wide variety of trucks in terms of route, geography, vehicle type, and use case. In doing so, they have successfully called the trucking industry’s attention to effective, ready-for-market technologies, dispelling common misunderstandings and myths.
Beyond the Run on Less events, NACFE also issues confidence reports on established technologies and guidance reports on emerging technologies, as well as hosting regular trainings and workshops to help fleet owners and other relevant stakeholders make informed decisions.
Avoiding steel emissions by reducing our need for steel
Steel is one of the largest greenhouse gas emitters in heavy industry. Accordingly, new policies have been advanced in the United States, the European Union, India, and Australia to accelerate “green” steel production through direct subsidies, export credits and loans, green procurement, and penalties for emitting CO2 from traditional steelmaking. While green steel is an important way to reduce greenhouse gas emissions, there are other potent levers to reduce the emissions associated with iron and steel while respecting planetary boundaries. Starting with the end in mind, let’s consider how and why steel is used and assess a fuller range of efficiency solutions — including demand-side levers, materials efficiency, and circularity.
How does steel contribute to our well-being? The biggest uses of steel are in buildings, vehicle manufacturing, and transport infrastructure. So, how could our cities, homes, and mobility evolve to affect the demand for steel?
Reducing Steel Demand
How much steel do we need? Potentially only half of what we think.
One study concludes that global energy demand for steel production could be 60 percent lower than in a business-as-usual scenario in 2050 (Exhibit 6) when taking full advantage of demand-side approaches such as the following:
- Material efficiency focuses on providing the same material services with fewer materials. For example, smaller cars use less steel and other materials than SUVs or pickup trucks, but they can equally fulfill day-to-day needs in most cases.
- Material substitution aims to substitute away from energy-intensive materials such as steel and concrete. For example, steel can be replaced with bio-based structural materials or advanced synthetic materials.
- Material circularity reduces energy and resources by using recycled materials. For instance, producing a unit of recycled steel uses only 10–25 percent as much energy as producing virgin steel. This is primarily because virgin steel production requires an extra step of processing (iron ore reduction) that is not required when recycling scrap steel.
Separately, Mission Possible Partnership’s Steel Transition Strategy, endorsed by major global steel manufacturers, concluded that steel demand could be reduced by 41 percent in 2050 via circularity, productivity of use, and material efficiency alone.
Green Steel Production
Where primary steel production cannot be avoided, novel iron reduction and steelmaking methods can substantially reduce emissions. Best known today is hydrogen-based direct reduction, which uses green hydrogen produced from renewable energy, but there are other emerging technologies such as molten oxide electrolysis, electrowinning, and smelting via hydrogen plasma or lasers. These methods are still very energy-intensive — highlighting the importance of other efficiency measures alongside continued improvements of these methods themselves — but they can be fully powered by renewable electricity, so they offer the best path forward to reduce emissions from primary steel production.
Exhibit 6. Impacts of End-Use Efficiency Measures on Global Energy Demand from Steel Production in 2050
Industrial Clusters and Supply Chains
The development of industrial hubs and clusters offers additional system-level opportunities to reduce energy demand and emissions from steel. For example, industrial clusters could up-level energy efficiency by repurposing waste heat and materials, sharing infrastructure, and other synergies. The energy savings from such “industrial symbiosis” across steel, cement, aluminum, and paper production could be as high as 18 percent.
Industrial hubs will become critical as steel production shifts to green hydrogen. Many planned green steel factories will be sited near hydrogen hubs. For example, the Pilbara Hydrogen Hub in Australia will colocate green steel facilities with hydrogen production and export facilities. This can enable new modes of industrial symbiosis around green hydrogen, as well as create opportunities for cost and energy savings in transporting steel, as explained in the box below.
Reimagining the Steel Value ChainThe Green Iron Corridors project, led by RMI and the Green Hydrogen Catapult, envisions a radical restructuring of the steel value chain that will enable rapid growth of green steel while delivering significant cost and energy savings.
Traditionally, steel has been produced in large integrated facilities that include both the ironmaking and steelmaking process. This means that steel production is currently sited where coal and iron ore — both key inputs for traditional steelmaking — are cheap.
However, with the rise of green steel made using green hydrogen, the calculus changes. The Green Iron Corridors project proposes splitting up the ironmaking and steelmaking processes, with ironmaking sited in locations with abundant iron ore and renewable energy, such as Australia, and steelmaking in locations with existing manufacturing capabilities and strong demand markets, such as Germany.
Transforming this value chain not only facilitates the growth of green steel, but also improves energy efficiency by avoiding unnecessary transport. Rather than shipping both iron ore and hydrogen (via an ammonia carrier), importing a finished green iron product could save up to 3.1 TWh of total electricity use for a commercial-scale plant producing two million tons of steel per year (even considering the necessary reheating step to produce steel from cold iron). Producing green hydrogen in renewable-rich regions is also more infrastructure-efficient than doing so in traditional demand centers: completely replacing the EU’s nearly 100 million tons of traditional, integrated, carbon-intensive production with green production would require 70 GW less renewable capacity in Western Australia than it would in Germany.
Multiple benefits: Lower emissions, costs, and pollution
The energy efficiency opportunities described here align with a common pattern. In each case, significant savings can come from technology change — from green steel to electrification in buildings, cars, and freight. And in each example, energy end-use efficiency can bring further savings while facilitating the adoption of new technologies.
However, energy end-use efficiency is also powerful because it has cross-cutting benefits across sectors. Exhibit 7 summarizes many of the efficiency measures we’ve highlighted, emphasizing the interconnections in yellow. Urban land use and transportation policies and planning should go hand in hand, with efficiency implications for buildings, transportation, and even the steel sector and beyond — since fewer cars means less material demand for car-related infrastructure. Similarly, material efficiency, substitution, and circularity practices can reduce demand for steel while relieving freight burdens. Less freight, in turn, contributes to less traffic — further reducing demand for transportation infrastructure.
The benefits of these efficiency measures extend beyond energy savings to human well-being, as we discuss in a separate article. Fewer cars means less traffic, which means improved air quality and lower risk of traffic accidents. More walking and cycling mean better health outcomes. Passive housing design can increase household resilience to severe weather events.
In short, energy end-use efficiency measures are systemic leverage points where one intervention can lead to broader benefits for human well-being — which is why, after all, we use energy in the first place.
Exhibit 7. The Cross-Cutting Benefits of Energy End-Use Efficiency Measures

Delivering on global goals to double energy efficiency improvement rates will not only require major technology transitions in key sectors, but also better planning, design, operation, system integration, and circularity. As Amory Lovins writes, “saving energy is like eating a lobster: if you extract only the large chunks of meat from the tail and claws and throw away the rest, you will miss a comparable amount of tasty morsels tucked in crevices.”
These changes, in turn, depend on a diverse range of actors including policymakers, private companies, civil society, and consumers themselves, supported by a social norm that values efficiency and sufficiency. Establishing this kind of awareness provides an ethic of care that inspires and aligns actions at many different levels, including strengthening support for government policies and motivating corporate energy efficiency efforts.
Valuing EfficiencyThe idea of frugality may seem passé in the context of our supercharged, consumption-oriented global economy, but some leaders today are invoking this value as a key part of the path to greater well-being. Researchers working on energy and climate have chosen the more analytical term high-with-low to describe pathways to sustainability that center demand-side solutions for high well-being with low resource demand. These pathways achieve high levels of human development while keeping human resource footprints within planetary boundaries. Research shows that while demand-side solutions are generally underemphasized in the analysis of energy transition pathways, high-with-low scenarios show great promise for delivering a sustainable future while mitigating the worst consequences of climate change.
Is it possible to revive efficiency as a social norm? Values such as efficiency and frugality have deep and cross-cultural roots. Confucius promoted moderation as central to leading a virtuous life; he believed that practicing frugality was a way to cultivate moral character, humility, and responsibility. Mohandas Gandhi said, “The world has enough for everyone’s need, but not enough for everyone’s greed” and encouraged all people to “live simply so that others may simply live.” In the early history of the United States, Benjamin Franklin coined the phrase “a penny saved is a penny earned,” and he made the ethic of frugality a constant theme of his writings in Poor Richard’s Almanac, published to the 13 colonies for over 25 years from 1732 to 1758. “Without industry and frugality nothing will do; with them, everything,” Franklin wrote.
Given the diversity of actors involved in delivering energy efficiency, refreshing the societal values of efficiency and frugality can be an important force to reinforce these actions. Is a social tipping point in this direction possible?
Here are some examples of existing actions to highlight the energy efficiency resource — and the benefits of unlocking it:
- Global: Leaders of 197 nations plus the European Union signed a Framework Convention at COP28 setting a goal to double the rate of energy efficiency improvement between the time of the agreement and 2030. The agreement raised the profile of energy efficiency among national governments, energy and climate experts, and the media. Other global efforts have aligned with this goal, including Mission Efficiency — an alliance of partners from civil society and public- and private-sector institutions urging world leaders to unlock the energy efficiency investment that is crucial to meeting climate targets.
- National: Many countries have adopted national goals and/or launched campaigns to promote energy efficiency at a national or multinational level. These include India’s Lifestyles for Environment (LiFE), a mass movement championed by Prime Minister Modi to nudge individual and community action to protect and preserve the environment. “What is needed today is mindful and deliberate utilization, instead of mindless and destructive consumption,” says Modi. Today, over 1 million schools in India are connected to eco-clubs aimed at raising awareness about sustainable lifestyles. Other awareness campaigns include the EU’s NUDGE project, which implements and evaluates different behavioral interventions for energy efficiency, paving the way for new policies and increased consumer awareness, and Japan’s “Cool Biz” campaign that encourages weather-appropriate attire for office workers. Other countries such as New Zealand and Bhutan take a more holistic approach to encourage an energy efficient — or sufficient — lifestyle by encouraging a focus on well-being.
- City and community: Many cities and communities around the world have emphasized energy efficiency in their planning, policies, and outreach. One example of a community with an integrated approach to energy and resource efficiency is Palava City, which was mentioned above as an example of walkable urban design, with parallel efforts in energy, materials, and water efficiency. The city is designed to be a “living laboratory” for continuously refining and improving sustainability practices. Another example is the Vauban district of Freiburg, Germany, where every building has rooftop solar and many are built according to passive design principles. The city is built around walking, biking, and a tramway, and it has a sustainable waste management system.
- Corporate and industry: Businesses have established numerous coalitions to increase awareness of energy efficiency opportunities and accelerate implementation of solutions. For example, EP100 is a global corporate energy efficiency initiative, led by Climate Group, bringing together more than 125 ambitious businesses committed to improving their energy efficiency. EP100 members are committed to doubling their energy productivity, rolling out energy management systems, or achieving net-zero carbon buildings. By making public commitments, and driving toward those targets, companies can show climate leadership and send a powerful signal to policymakers and other businesses about the enormous potential of energy efficiency.
Acknowledgments
Special thanks to Ari Kahn, Kaitlyn Ramirez, Mike Roeth, Eva Rosenbloom, Lucas Toffoli, Brett Webster, Rachel Wilmoth, and Nick Yavorsky for their contributions.
The authors acknowledge support from the Energy Demand changes Induced by Technological and Social innovations (EDITS) project, which is part of the initiative coordinated by the Research Institute of Innovative Technology for the Earth (RITE) and the International Institute for Applied Systems Analysis (IIASA), funded by the Ministry of Economy, Trade, and Industry (METI), Japan.
Focusing on the desired end use places purposes and application before equipment, efficiency before supply, passive before active, simple before complex.
Let’s take a step back and think about why we use energy in the first place. In many cases, the goal is to fulfill human needs — ranging from physiological needs such as food, hygiene, and shelter, all the way to social needs fulfilled by education, employment, and recreation. We rely on energy services to meet many of these needs. Energy services are functions performed using energy in order to achieve desired outcomes such as maintaining thermal comfort, cooking and preserving food, heating water, and getting to the places we need to be.
Energy services are provided by technologies such as cookstoves; heating, ventilation, and air conditioning (HVAC) systems; and vehicles. All these technologies generate useful energy (the energy that goes toward the desired service, e.g., heat, radiant energy, kinetic energy) using final energy (the energy a consumer purchases, e.g., metered electricity, gasoline). Final energy is provided by primary energy sources such as the sun, the wind, and fossil fuels.
Exhibit 2. Examples of Relationships between Human Well-Being, Energy Services, and Energy Technologies
Traditional energy efficiency programs often focus on specific product and appliance upgrades. These programs aim to minimize conversion losses from final energy to useful energy, such as the waste heat from a car’s combustion engine. As important as these measures are, they center on a narrow definition of energy efficiency.
When we focus on human needs, we start to see that the energy efficiency solution space extends well beyond better machines to include changing the way we fulfill human needs while generating significant energy and cost savings. Examples include walkable neighborhoods, better cycling infrastructure, passive heating and cooling, “daylighting” our buildings, and much more. These solutions are what we call energy end-use efficiency.
Electrification offers an opportunity to radically improve efficiency in energy technologies, far beyond conventional appliance upgrades. For example, upgrading your gas furnace could reduce energy use by about 10 percent — but switching to a heat pump could reduce energy use by as much as 30–50 percent. At the same time, electrification comes with certain challenges, notably electricity load growth and affordability for users, and it only taps part of the economically available efficiency resource.
This is where energy end-use efficiency can and should play an important role. When we reduce our reliance on energy services, we also reduce the size or number of energy technologies we need. This means an easier and more affordable path to electrification: we need fewer EVs to satisfy a community’s mobility needs, and smaller — more affordable — heat pumps to satisfy people’s thermal comfort needs.
Fewer and smaller electrification technologies will also help to manage electricity demand. Again, electrification technologies are already far more efficient than fossil-based alternatives and would lessen total energy use on their own. But energy end-use efficiency takes these savings even further because the effects compound. As we show in the sector examples below, energy end-use efficiency and electrification combined can reduce overall energy demand by 50–75 percent. These savings can play a huge role in reducing burdens on the grid and easing the transition to a renewable energy future.
Attaining Thermal Comfort with Less Heating and Cooling Energy Use
Why do we use energy in buildings? One of the biggest reasons is to provide heating and cooling services. From a human perspective, the ultimate objective is to attain thermal comfort: we don’t want to be too hot, or too cold, in the buildings where we live or work.
A systems thinking approach to energy efficiency in heating and cooling entails consideration of usage, design, construction (or retrofit), and operation to identify the least-cost solutions to deliver high levels of well-being. These system measures can shrink the need for mechanical heating and cooling services and, in turn, the size and cost of heat pumps or other equipment required.
Mixed-Use, Human-Scale Development
One solution is simply to use floor space more efficiently. This is key for rapidly growing cities in the Global South, where most of the building stock is yet to be built, but it is just as relevant for growing metropolitan regions in the Global North that can achieve major impact through zoning reforms.
Multifamily homes save about 50 percent of a household’s energy compared with single-family detached homes, due to their density and shared walls, which reduce heat gain or loss. Market studies find a growing demand for compact development, especially among households without children that prioritize access and location over unit size.
Compact urban development also opens the door for district heating and cooling systems, which are most viable in high-density, mixed-use areas. District systems can reduce primary energy consumption by 30–50 percent by unlocking more efficient sources of heating and cooling — such as waste heat from data centers, large-scale heat pumps, or geothermal energy — which may be less feasible at the individual household or building level.
Passive Housing Design
There are many ways to maintain comfortable temperatures without mechanical heating or cooling. The most common example is a high-performance building envelope. In cooler climates, this means improving insulation and airtightness in the walls, roof, and foundation to minimize heat loss. Such envelope improvements also help retain cool air in warmer seasons. According to RMI’s Carbon-Free Buildings team, basic targeted weatherization, air sealing, and added insulation can typically generate energy savings of about 10–30 percent. Deep energy retrofits, which include comprehensive air sealing, added insulation, and high-performance windows and/or doors, can generate energy savings of 60–80 percent.
In hotter climates, there is an additional objective to minimize heat absorption and even emit heat. Cool roofs designed to reflect, rather than absorb, sunlight can passively reduce the internal temperature of the building and lessen the need for air conditioning. The most advanced cool roof materials don’t just reflect heat — they actively remove it from the building, lowering indoor temperatures by up to 10°C. This translates to energy savings of 20–70 percent, depending on the building type and climate.
Planting trees in urban areas can also help reduce the risk of high heat events that strain energy systems and harm human health. Trees mitigate the urban heat island effect primarily through shading and transpiration. In India, real estate developers combined various measures including building orientation, shading, and landscaping to achieve an impressive 7°C reduction in local land surface temperatures in a new community near Mumbai.
At the human level, dressing appropriately for the weather can reduce overreliance on heaters and air conditioners. Thermal variety within comfortable temperatures offers additional benefits for the human sensory experience.
Heat Pumps and Superefficient Air Conditioners
Lower demand for mechanical heating and cooling means that the same building can run on a smaller HVAC system, thereby making the investment more affordable. It also reduces the electricity demand, easing the transition to a fully renewable grid.
Heat pumps provide a leap in efficiency relative to fossil fuel heating systems and conventional compressor-based air conditioning. Because heat pumps move heat — as opposed to fossil fuel furnaces or heaters that rely on combustion to generate heat — they can produce over four times as much heat energy as they consume in electricity, saving up to half the total energy while producing zero direct emissions. Switching from fossil fuels also reduces indoor air pollution and removes potential risks of carbon monoxide poisoning and gas leaks.
Meanwhile, manufacturers have demonstrated the feasibility of superefficient air conditioners that have five times less climate impact than standard air conditioners currently available on the market.
Exhibit 3. Impacts of End-Use Efficiency Measures on Global Energy Demand from Residential Buildings in 2050
Scaling Deep Energy Retrofits in Massachusetts and California
Despite the potential of building envelope improvements, implementing retrofits often proves challenging. Deep energy retrofits require significant up-front investment, can be highly disruptive to occupants, and require owners to navigate through conflicting guidance, fragmented financing, and administrative burdens.
Inspired by innovative whole-building retrofit programs in Europe, RMI launched a market facilitation initiative for multifamily homes in Massachusetts and California to address these challenges, streamline deep energy retrofits, and demonstrate a scalable model. To make retrofits accessible for as many people as possible, particularly in historically marginalized communities, this initiative aims to facilitate a retrofit pipeline, support owners through the retrofitting process, and showcase innovative retrofit packages for common building typologies that drastically improve building performance. These efforts build market capacity and standardize the retrofit process while simultaneously aggregating demand from multifamily building owners with similar typologies.
The retrofit approach varies across building typologies and may include easily installable prefabricated insulated facade panels, insulated roof panels with solar, combined heat pump space conditioning and water heating systems, and electric appliances. Having a standardized program and common retrofit approach for each typology helps facilitate the retrofit process in several ways, including lowering information barriers for owners and shortening installation time, which reduces disruption for occupants. Standardization also makes it easier to aggregate demand and mitigate perceived risks, bringing down the price of key components.
In Massachusetts, RMI aims to have 1,000 multifamily apartment units under renovation by the end of 2024. Meanwhile, in California, RMI is demonstrating retrofit solutions on over 300,000 square feet of pilots. RMI provides further support for owners to help ease administrative and financial burdens through a streamlined application process, decarbonization assessments, assistance finding financing options, matching owners with contractors, and more.
Getting everyone to their destinations with less energy
Cars have been a primary target of energy and climate policy for many decades. Though policy success has varied, average vehicle efficiency has gradually improved, even as the number of cars on the road and the number of miles driven have continued to increase globally.
How can we radically improve energy efficiency in the mobility sector? The answer starts with land -use solutions that minimize vehicle dependence altogether, coupled with better public transit systems to enable the use of less energy-intensive transportation options such as trains and buses. Where private car usage remains necessary, electric vehicles — especially smaller, lighter ones — can offer a leap forward in efficiency.
Mixed-Use, Human-Scale Development
Strategic land use can help avoid vehicle dependence altogether by bringing key services and activities closer to one’s home. In other words, people can walk, bike, and move to places without the use of a motorized vehicle. Studies by RMI consistently find that reforms to land use policy in the United States, whether at the state or city level, can lead to per capita reductions in vehicle miles traveled (VMT) of around 10 percent.
Mixed-use and human-scale development are often attributed to urban areas but are also possible in other settings: think of the quintessential small town “Main Street” found across rural and suburban areas of the United States, or the recent burgeoning of walkable exurban downtowns. Walking and biking also offer additional health benefits, as in New York City, where bike lanes gave residents an extra year of healthy life at far lower cost than most health interventions.
Meanwhile, some private developers, like India’s Lodha Group, are choosing to develop new walkable, bikeable communities based on the market pull for this way of living. Lodha’s Palava City development, near Mumbai, follows a 5-10-15 neighborhood planning principle. This approach prioritizes having daily necessities like play areas, convenience retail, and schools within a five-minute walking distance, recreational and entertainment venues within a 10-minute walk, and necessities like healthcare facilities, banks, and offices within a 15-minute walk.
Public Transit Systems
Better transit systems can incentivize modal shifts by providing a more attractive alternative to private cars. What do “better” transit systems look like? Riders prize higher service quality in the form of increased coverage of routes and frequency of service, as well as general comfort, speed (e.g., privileged access for buses on busy roadways), safety, payment convenience, and cleanliness. Offering a wide range of options, including buses and trains but also micromobility (shared bikes and scooters), can be helpful too. Like walking and biking, better public transit also offers additional health benefits as well as equity benefits such as increased job access.
A global study by the Institute for Transportation and Development Policy (ITDP) finds that a range of measures can reduce private car activity by 50 percent, nearly halving overall energy demand from passenger transport (Exhibit 4). These measures combine transit-oriented urban development with government policies at all levels that are committed to more bicycling and pedestrian infrastructure, public transit, sustainable land use, and traffic reduction.
Electric Vehicles
Today, transformational change is afoot with the advent of competitively priced electric vehicles that are typically two to four times as efficient as cars with internal combustion engines. Because they are so efficient, EVs can reduce vehicle energy demand by 32–70 percent, depending on the source of electricity. Thus, the exponential growth of electric vehicles globally will revolutionize the efficiency of the mobility sector.
Combining electrification with the aforementioned end-use efficiency measures can bring a 67 percent reduction in energy demand from urban passenger transport, according to the same ITDP study.
Exhibit 4. Impacts of End-Use Efficiency Measures on Global Energy Demand from Urban Passenger Transport in 2050
Smaller, Lighter Cars
Basic physics dictates that heavier things require more energy to move. The weight of cars can be reduced by using lightweight materials such as high-strength steel, magnesium alloys, aluminum alloys, carbon fiber, and polymer composites. Experts estimate that vehicle weight can be lowered by 35 percent at reasonable cost, which could reduce fuel consumption by 12–20 percent with no trade-offs in terms of safety or performance. The cost of these lightweight materials can be offset at the point of manufacturing by the lower price of smaller motors and engines, even before considering fuel cost savings for the consumer.
Beyond materials, another key factor is size. Bigger cars weigh more and require more energy to move. Cars have grown steadily over the years, driven by the fact that vehicle models get bigger with every design generation, as well as by an overall market shift from compact cars and sedans to SUVs. The impact of car size is not to be underestimated: the Global Fuel Economy Initiative finds that without the shift to SUVs, more than half of vehicle weight increases could have been avoided between 2010 and 2022.
Making cars smaller and lighter will also ease the transition to electric cars. A smaller and lighter electric car needs a smaller battery, which makes it more affordable and faster to charge. Smaller and lighter cars will also improve road safety: in the event of a collision, larger, heavier cars increase the risk of severe injury or death for cyclists, pedestrians, and drivers of smaller vehicles, while offering diminishing returns in terms of safety for the driver of the larger vehicle.
Urban Transportation in Three Cities: Tokyo, Paris, and Bogotá
Tokyo is renowned for its public transportation system. The coverage of the subway system, and the frequency at which trains run, far eclipses most American cities. You’re never more than 5 to 10 minutes from a subway station, and trains run about every 5 minutes (or even less, depending on the line). On top of that, they are clean and rarely late.
Because mixed-use development is the norm in most areas of Tokyo, most people do not need cars in their daily lives. About a quarter of Tokyo residents do not have driver’s licenses. Only 50 percent own a car — down from 60 percent a decade ago — and one-third of those are compact or Kei cars. Less than 3 percent own trucks or vans. All in all, this means that transportation energy use in Tokyo is just 18 GJ per capita, compared with 47 GJ per capita in New York, while helping people get to the places they want or need to be.
It may feel challenging to transform cities at this scale, but it is possible, and we have seen it happen.
Paris began its transformation into a “100 percent bikeable city” in 2015 with the Cycling Plan (Plan Vélo). More than 70 percent of the city’s parking spaces were removed and replaced with pedestrian and cycling infrastructure. More trips are now made by bike than by car — both within the city as well as for trips between the suburbs and the city center. In the past two decades, driving within Paris has decreased by 60 percent, mirrored by a comparable increase in walking, bicycling, and public transit.
Bogotá went through a similar change two decades ago, though its experience since offers a more cautionary tale. In the early 2000s Bogotá developed the TransMilenio, a rapid bus system, to address the city’s rapidly growing population and deteriorating transportation infrastructure. By 2012, TransMilenio operated 12 lines totaling 112 kilometers, with around 1,500 buses serving 1.5 million passenger journeys each day. Initially, it was hailed as a success, reducing congestion and improving air quality, passenger safety, and accessibility. Today, however, the New York Times calls the TransMilenio an “embattled institution.” Political turnover shifted budget priorities, which led to a decline in service despite further increases to ridership: buses were not replaced as they aged, and promised route extensions were never delivered. To truly enable optimal transportation efficiency, good urban development must be an ongoing priority with sustained investment.
Fulfilling our material needs with less freight movement
We rely on a great number of material goods in our lives, and the freight industry plays a critical role in transporting these materials and goods to where they are needed. Yet at the same time, freight transportation makes up 8 percent of global greenhouse gas emissions, a majority of which comes from road vehicles such as trucks.
The way to improve trucking efficiency is by replacing inefficient trucks with more efficient ones, right? Certainly, the emergence of cost-competitive electric trucks will be a major help. But while more efficient trucks are a big part of the solution, focusing on trucks alone obscures many other opportunities for efficiency improvement in the freight transportation system, ranging from driver behavior to the overall shape of our future economy. Together, these other levers could account for a large share of the overall savings opportunity.
Material Demand Reduction
The starting point for reducing trucking emissions is not in the trucking sector, or even the larger freight sector, but rather in basic assumptions about our future economy. One possibility comes from the Low Energy Demand (LED) scenario developed by the International Institute for Applied Systems Analysis (IIASA), which provides a widely referenced benchmark for a low-energy-demand economy. LED assesses how the quantities and types of services drive supply and requirements for energy, materials, and land. Solutions include familiar measures like product lightweighting (e.g., smaller, lighter cars), fewer cars, and more efficient use of residential floorspace — as well as additional measures such as increased product longevity, sharing and service business models, reduced loss and waste, and increased material efficiency. All these measures reduce the quantity and volume of goods that must be moved, while maintaining or improving human well-being.
There are solutions across sectors, including the freight system itself. For example, Walmart’s whole-systems approach has included working with supply chain partners to lessen the volume of packaging, lighten certain cargo, improve truck loading techniques, and more.
Combined with modal shifts to rail, which we discuss below, LED shows that freight activity can be reduced to less than half of business-as-usual scenarios such as the International Energy Agency’s Stated Policies Scenario, or STEPS (Exhibit 5).
Freight Network Improvements
In parallel with material demand reduction, there are additional opportunities to reduce trucking demand through changes to the overall freight system.
Notably, shifting freight from truck to rail can lead to significant energy and cost savings, as trucks consume nearly three times more energy per ton-km as compared with rail. While this sounds straightforward, facilitating this modal shift is complex — often because today’s long-distance rail freight routes require high shipment densities to be profitable. Solutions include the integration of long-distance and short-line rail, aided by the build-out of smaller, low-cost intermodal hubs (for rail-to-truck transfer), or logistics hubs that consolidate freight volumes across multiple firms and industries. These hubs can also help improve route optimization and reduce the distance that trucks travel empty or below capacity.
Another solution is regional trucking, which can enable more direct routes and reduce miles traveled by as much as a third when combined with practices such as local warehousing and relay trucking — and can even reduce idling time.
Battery Electric Trucks and E-Cargo Bikes
By enabling shorter, simpler trucking routes, these network improvements go hand-in-hand with electrification. Electric trucks are currently better suited to shorter routes and require reliable charging availability, though this may change as battery technologies improve. Electric trucks elevate efficiency because they can be three to seven times more efficient than diesel trucks. Depending on the electricity source, this translates to a 60–80 percent reduction in emissions.
For last-mile deliveries, e-cargo bikes and electric three-wheelers provide significant energy savings, including in many emerging market economies. E-cargo bikes, for example, use 90 percent less energy per kilometer compared with an electric van, and they provide other benefits such as improved pedestrian safety and reduced congestion.
Driver Performance
Finally, where trucking cannot be reduced or avoided, we can improve efficiency by improving driver behavior. The fuel economy of trucks is greatly influenced by factors that drivers can control, such as speed, acceleration, braking, gear selection, idling, and more. Research shows that drivers can affect fuel efficiency by more than 20 percent. There are many ways to improve driver performance, including via training, incentives, and real-time driver feedback through telematic systems.
Exhibit 5. Impacts of End-Use Efficiency Measures on Global Energy Demand from Freight Trucking in 2050
Cultivating the Efficiency Mindset in the Trucking Industry The North American Council on Freight Efficiency (NACFE) is a fuel-agnostic organization focused on promoting the development and adoption of energy efficiency in the freight industry. NACFE shows how industry partners can help to increase awareness about efficiency-related cost savings, share and disseminate learnings about best practices, and create an industry-wide culture of continuous improvement.
Since 2017, in collaboration with RMI, NACFE has hosted four high-level demonstration events (NACFE Run on Less) aimed at showcasing the feasibility and impact of various trucking efficiency measures. The first two events highlighted the full savings potential from existing technologies, including for long-haul trucks and regional haul trucks. The 2021 and 2023 Run on Less events focused on battery electric trucks and truck charging depots, showcasing how fleets can maximize their benefits and scalability.
Each event has incorporated timely issues for the trucking industry, such as the growing regional haul market and the falling costs and weights of batteries. In addition, all demonstrations have involved a wide variety of trucks in terms of route, geography, vehicle type, and use case. In doing so, they have successfully called the trucking industry’s attention to effective, ready-for-market technologies, dispelling common misunderstandings and myths.
Beyond the Run on Less events, NACFE also issues confidence reports on established technologies and guidance reports on emerging technologies, as well as hosting regular trainings and workshops to help fleet owners and other relevant stakeholders make informed decisions.
Avoiding steel emissions by reducing our need for steel
Steel is one of the largest greenhouse gas emitters in heavy industry. Accordingly, new policies have been advanced in the United States, the European Union, India, and Australia to accelerate “green” steel production through direct subsidies, export credits and loans, green procurement, and penalties for emitting CO2 from traditional steelmaking. While green steel is an important way to reduce greenhouse gas emissions, there are other potent levers to reduce the emissions associated with iron and steel while respecting planetary boundaries. Starting with the end in mind, let’s consider how and why steel is used and assess a fuller range of efficiency solutions — including demand-side levers, materials efficiency, and circularity.
How does steel contribute to our well-being? The biggest uses of steel are in buildings, vehicle manufacturing, and transport infrastructure. So, how could our cities, homes, and mobility evolve to affect the demand for steel?
Reducing Steel Demand
How much steel do we need? Potentially only half of what we think.
One study concludes that global energy demand for steel production could be 60 percent lower than in a business-as-usual scenario in 2050 (Exhibit 6) when taking full advantage of demand-side approaches such as the following:
- Material efficiency focuses on providing the same material services with fewer materials. For example, smaller cars use less steel and other materials than SUVs or pickup trucks, but they can equally fulfill day-to-day needs in most cases.
- Material substitution aims to substitute away from energy-intensive materials such as steel and concrete. For example, steel can be replaced with bio-based structural materials or advanced synthetic materials.
- Material circularity reduces energy and resources by using recycled materials. For instance, producing a unit of recycled steel uses only 10–25 percent as much energy as producing virgin steel. This is primarily because virgin steel production requires an extra step of processing (iron ore reduction) that is not required when recycling scrap steel.
Separately, Mission Possible Partnership’s Steel Transition Strategy, endorsed by major global steel manufacturers, concluded that steel demand could be reduced by 41 percent in 2050 via circularity, productivity of use, and material efficiency alone.
Green Steel Production
Where primary steel production cannot be avoided, novel iron reduction and steelmaking methods can substantially reduce emissions. Best known today is hydrogen-based direct reduction, which uses green hydrogen produced from renewable energy, but there are other emerging technologies such as molten oxide electrolysis, electrowinning, and smelting via hydrogen plasma or lasers. These methods are still very energy-intensive — highlighting the importance of other efficiency measures alongside continued improvements of these methods themselves — but they can be fully powered by renewable electricity, so they offer the best path forward to reduce emissions from primary steel production.
Exhibit 6. Impacts of End-Use Efficiency Measures on Global Energy Demand from Steel Production in 2050
Industrial Clusters and Supply Chains
The development of industrial hubs and clusters offers additional system-level opportunities to reduce energy demand and emissions from steel. For example, industrial clusters could up-level energy efficiency by repurposing waste heat and materials, sharing infrastructure, and other synergies. The energy savings from such “industrial symbiosis” across steel, cement, aluminum, and paper production could be as high as 18 percent.
Industrial hubs will become critical as steel production shifts to green hydrogen. Many planned green steel factories will be sited near hydrogen hubs. For example, the Pilbara Hydrogen Hub in Australia will colocate green steel facilities with hydrogen production and export facilities. This can enable new modes of industrial symbiosis around green hydrogen, as well as create opportunities for cost and energy savings in transporting steel, as explained in the box below.
Reimagining the Steel Value ChainThe Green Iron Corridors project, led by RMI and the Green Hydrogen Catapult, envisions a radical restructuring of the steel value chain that will enable rapid growth of green steel while delivering significant cost and energy savings.
Traditionally, steel has been produced in large integrated facilities that include both the ironmaking and steelmaking process. This means that steel production is currently sited where coal and iron ore — both key inputs for traditional steelmaking — are cheap.
However, with the rise of green steel made using green hydrogen, the calculus changes. The Green Iron Corridors project proposes splitting up the ironmaking and steelmaking processes, with ironmaking sited in locations with abundant iron ore and renewable energy, such as Australia, and steelmaking in locations with existing manufacturing capabilities and strong demand markets, such as Germany.
Transforming this value chain not only facilitates the growth of green steel, but also improves energy efficiency by avoiding unnecessary transport. Rather than shipping both iron ore and hydrogen (via an ammonia carrier), importing a finished green iron product could save up to 3.1 TWh of total electricity use for a commercial-scale plant producing two million tons of steel per year (even considering the necessary reheating step to produce steel from cold iron). Producing green hydrogen in renewable-rich regions is also more infrastructure-efficient than doing so in traditional demand centers: completely replacing the EU’s nearly 100 million tons of traditional, integrated, carbon-intensive production with green production would require 70 GW less renewable capacity in Western Australia than it would in Germany.
Multiple benefits: Lower emissions, costs, and pollution
The energy efficiency opportunities described here align with a common pattern. In each case, significant savings can come from technology change — from green steel to electrification in buildings, cars, and freight. And in each example, energy end-use efficiency can bring further savings while facilitating the adoption of new technologies.
However, energy end-use efficiency is also powerful because it has cross-cutting benefits across sectors. Exhibit 7 summarizes many of the efficiency measures we’ve highlighted, emphasizing the interconnections in yellow. Urban land use and transportation policies and planning should go hand in hand, with efficiency implications for buildings, transportation, and even the steel sector and beyond — since fewer cars means less material demand for car-related infrastructure. Similarly, material efficiency, substitution, and circularity practices can reduce demand for steel while relieving freight burdens. Less freight, in turn, contributes to less traffic — further reducing demand for transportation infrastructure.
The benefits of these efficiency measures extend beyond energy savings to human well-being, as we discuss in a separate article. Fewer cars means less traffic, which means improved air quality and lower risk of traffic accidents. More walking and cycling mean better health outcomes. Passive housing design can increase household resilience to severe weather events.
In short, energy end-use efficiency measures are systemic leverage points where one intervention can lead to broader benefits for human well-being — which is why, after all, we use energy in the first place.
Exhibit 7. The Cross-Cutting Benefits of Energy End-Use Efficiency Measures

Delivering on global goals to double energy efficiency improvement rates will not only require major technology transitions in key sectors, but also better planning, design, operation, system integration, and circularity. As Amory Lovins writes, “saving energy is like eating a lobster: if you extract only the large chunks of meat from the tail and claws and throw away the rest, you will miss a comparable amount of tasty morsels tucked in crevices.”
These changes, in turn, depend on a diverse range of actors including policymakers, private companies, civil society, and consumers themselves, supported by a social norm that values efficiency and sufficiency. Establishing this kind of awareness provides an ethic of care that inspires and aligns actions at many different levels, including strengthening support for government policies and motivating corporate energy efficiency efforts.
Valuing EfficiencyThe idea of frugality may seem passé in the context of our supercharged, consumption-oriented global economy, but some leaders today are invoking this value as a key part of the path to greater well-being. Researchers working on energy and climate have chosen the more analytical term high-with-low to describe pathways to sustainability that center demand-side solutions for high well-being with low resource demand. These pathways achieve high levels of human development while keeping human resource footprints within planetary boundaries. Research shows that while demand-side solutions are generally underemphasized in the analysis of energy transition pathways, high-with-low scenarios show great promise for delivering a sustainable future while mitigating the worst consequences of climate change.
Is it possible to revive efficiency as a social norm? Values such as efficiency and frugality have deep and cross-cultural roots. Confucius promoted moderation as central to leading a virtuous life; he believed that practicing frugality was a way to cultivate moral character, humility, and responsibility. Mohandas Gandhi said, “The world has enough for everyone’s need, but not enough for everyone’s greed” and encouraged all people to “live simply so that others may simply live.” In the early history of the United States, Benjamin Franklin coined the phrase “a penny saved is a penny earned,” and he made the ethic of frugality a constant theme of his writings in Poor Richard’s Almanac, published to the 13 colonies for over 25 years from 1732 to 1758. “Without industry and frugality nothing will do; with them, everything,” Franklin wrote.
Given the diversity of actors involved in delivering energy efficiency, refreshing the societal values of efficiency and frugality can be an important force to reinforce these actions. Is a social tipping point in this direction possible?
Here are some examples of existing actions to highlight the energy efficiency resource — and the benefits of unlocking it:
- Global: Leaders of 197 nations plus the European Union signed a Framework Convention at COP28 setting a goal to double the rate of energy efficiency improvement between the time of the agreement and 2030. The agreement raised the profile of energy efficiency among national governments, energy and climate experts, and the media. Other global efforts have aligned with this goal, including Mission Efficiency — an alliance of partners from civil society and public- and private-sector institutions urging world leaders to unlock the energy efficiency investment that is crucial to meeting climate targets.
- National: Many countries have adopted national goals and/or launched campaigns to promote energy efficiency at a national or multinational level. These include India’s Lifestyles for Environment (LiFE), a mass movement championed by Prime Minister Modi to nudge individual and community action to protect and preserve the environment. “What is needed today is mindful and deliberate utilization, instead of mindless and destructive consumption,” says Modi. Today, over 1 million schools in India are connected to eco-clubs aimed at raising awareness about sustainable lifestyles. Other awareness campaigns include the EU’s NUDGE project, which implements and evaluates different behavioral interventions for energy efficiency, paving the way for new policies and increased consumer awareness, and Japan’s “Cool Biz” campaign that encourages weather-appropriate attire for office workers. Other countries such as New Zealand and Bhutan take a more holistic approach to encourage an energy efficient — or sufficient — lifestyle by encouraging a focus on well-being.
- City and community: Many cities and communities around the world have emphasized energy efficiency in their planning, policies, and outreach. One example of a community with an integrated approach to energy and resource efficiency is Palava City, which was mentioned above as an example of walkable urban design, with parallel efforts in energy, materials, and water efficiency. The city is designed to be a “living laboratory” for continuously refining and improving sustainability practices. Another example is the Vauban district of Freiburg, Germany, where every building has rooftop solar and many are built according to passive design principles. The city is built around walking, biking, and a tramway, and it has a sustainable waste management system.
- Corporate and industry: Businesses have established numerous coalitions to increase awareness of energy efficiency opportunities and accelerate implementation of solutions. For example, EP100 is a global corporate energy efficiency initiative, led by Climate Group, bringing together more than 125 ambitious businesses committed to improving their energy efficiency. EP100 members are committed to doubling their energy productivity, rolling out energy management systems, or achieving net-zero carbon buildings. By making public commitments, and driving toward those targets, companies can show climate leadership and send a powerful signal to policymakers and other businesses about the enormous potential of energy efficiency.
Acknowledgments
Special thanks to Ari Kahn, Kaitlyn Ramirez, Mike Roeth, Eva Rosenbloom, Lucas Toffoli, Brett Webster, Rachel Wilmoth, and Nick Yavorsky for their contributions.
The authors acknowledge support from the Energy Demand changes Induced by Technological and Social innovations (EDITS) project, which is part of the initiative coordinated by the Research Institute of Innovative Technology for the Earth (RITE) and the International Institute for Applied Systems Analysis (IIASA), funded by the Ministry of Economy, Trade, and Industry (METI), Japan.
Cars have been a primary target of energy and climate policy for many decades. Though policy success has varied, average vehicle efficiency has gradually improved, even as the number of cars on the road and the number of miles driven have continued to increase globally.
How can we radically improve energy efficiency in the mobility sector? The answer starts with land -use solutions that minimize vehicle dependence altogether, coupled with better public transit systems to enable the use of less energy-intensive transportation options such as trains and buses. Where private car usage remains necessary, electric vehicles — especially smaller, lighter ones — can offer a leap forward in efficiency.
Mixed-Use, Human-Scale Development
Strategic land use can help avoid vehicle dependence altogether by bringing key services and activities closer to one’s home. In other words, people can walk, bike, and move to places without the use of a motorized vehicle. Studies by RMI consistently find that reforms to land use policy in the United States, whether at the state or city level, can lead to per capita reductions in vehicle miles traveled (VMT) of around 10 percent.
Mixed-use and human-scale development are often attributed to urban areas but are also possible in other settings: think of the quintessential small town “Main Street” found across rural and suburban areas of the United States, or the recent burgeoning of walkable exurban downtowns. Walking and biking also offer additional health benefits, as in New York City, where bike lanes gave residents an extra year of healthy life at far lower cost than most health interventions.
Meanwhile, some private developers, like India’s Lodha Group, are choosing to develop new walkable, bikeable communities based on the market pull for this way of living. Lodha’s Palava City development, near Mumbai, follows a 5-10-15 neighborhood planning principle. This approach prioritizes having daily necessities like play areas, convenience retail, and schools within a five-minute walking distance, recreational and entertainment venues within a 10-minute walk, and necessities like healthcare facilities, banks, and offices within a 15-minute walk.
Public Transit Systems
Better transit systems can incentivize modal shifts by providing a more attractive alternative to private cars. What do “better” transit systems look like? Riders prize higher service quality in the form of increased coverage of routes and frequency of service, as well as general comfort, speed (e.g., privileged access for buses on busy roadways), safety, payment convenience, and cleanliness. Offering a wide range of options, including buses and trains but also micromobility (shared bikes and scooters), can be helpful too. Like walking and biking, better public transit also offers additional health benefits as well as equity benefits such as increased job access.
A global study by the Institute for Transportation and Development Policy (ITDP) finds that a range of measures can reduce private car activity by 50 percent, nearly halving overall energy demand from passenger transport (Exhibit 4). These measures combine transit-oriented urban development with government policies at all levels that are committed to more bicycling and pedestrian infrastructure, public transit, sustainable land use, and traffic reduction.
Electric Vehicles
Today, transformational change is afoot with the advent of competitively priced electric vehicles that are typically two to four times as efficient as cars with internal combustion engines. Because they are so efficient, EVs can reduce vehicle energy demand by 32–70 percent, depending on the source of electricity. Thus, the exponential growth of electric vehicles globally will revolutionize the efficiency of the mobility sector.
Combining electrification with the aforementioned end-use efficiency measures can bring a 67 percent reduction in energy demand from urban passenger transport, according to the same ITDP study.
Exhibit 4. Impacts of End-Use Efficiency Measures on Global Energy Demand from Urban Passenger Transport in 2050
Smaller, Lighter Cars
Basic physics dictates that heavier things require more energy to move. The weight of cars can be reduced by using lightweight materials such as high-strength steel, magnesium alloys, aluminum alloys, carbon fiber, and polymer composites. Experts estimate that vehicle weight can be lowered by 35 percent at reasonable cost, which could reduce fuel consumption by 12–20 percent with no trade-offs in terms of safety or performance. The cost of these lightweight materials can be offset at the point of manufacturing by the lower price of smaller motors and engines, even before considering fuel cost savings for the consumer.
Beyond materials, another key factor is size. Bigger cars weigh more and require more energy to move. Cars have grown steadily over the years, driven by the fact that vehicle models get bigger with every design generation, as well as by an overall market shift from compact cars and sedans to SUVs. The impact of car size is not to be underestimated: the Global Fuel Economy Initiative finds that without the shift to SUVs, more than half of vehicle weight increases could have been avoided between 2010 and 2022.
Making cars smaller and lighter will also ease the transition to electric cars. A smaller and lighter electric car needs a smaller battery, which makes it more affordable and faster to charge. Smaller and lighter cars will also improve road safety: in the event of a collision, larger, heavier cars increase the risk of severe injury or death for cyclists, pedestrians, and drivers of smaller vehicles, while offering diminishing returns in terms of safety for the driver of the larger vehicle.
Urban Transportation in Three Cities: Tokyo, Paris, and Bogotá
Tokyo is renowned for its public transportation system. The coverage of the subway system, and the frequency at which trains run, far eclipses most American cities. You’re never more than 5 to 10 minutes from a subway station, and trains run about every 5 minutes (or even less, depending on the line). On top of that, they are clean and rarely late.
Because mixed-use development is the norm in most areas of Tokyo, most people do not need cars in their daily lives. About a quarter of Tokyo residents do not have driver’s licenses. Only 50 percent own a car — down from 60 percent a decade ago — and one-third of those are compact or Kei cars. Less than 3 percent own trucks or vans. All in all, this means that transportation energy use in Tokyo is just 18 GJ per capita, compared with 47 GJ per capita in New York, while helping people get to the places they want or need to be.
It may feel challenging to transform cities at this scale, but it is possible, and we have seen it happen.
Paris began its transformation into a “100 percent bikeable city” in 2015 with the Cycling Plan (Plan Vélo). More than 70 percent of the city’s parking spaces were removed and replaced with pedestrian and cycling infrastructure. More trips are now made by bike than by car — both within the city as well as for trips between the suburbs and the city center. In the past two decades, driving within Paris has decreased by 60 percent, mirrored by a comparable increase in walking, bicycling, and public transit.
Bogotá went through a similar change two decades ago, though its experience since offers a more cautionary tale. In the early 2000s Bogotá developed the TransMilenio, a rapid bus system, to address the city’s rapidly growing population and deteriorating transportation infrastructure. By 2012, TransMilenio operated 12 lines totaling 112 kilometers, with around 1,500 buses serving 1.5 million passenger journeys each day. Initially, it was hailed as a success, reducing congestion and improving air quality, passenger safety, and accessibility. Today, however, the New York Times calls the TransMilenio an “embattled institution.” Political turnover shifted budget priorities, which led to a decline in service despite further increases to ridership: buses were not replaced as they aged, and promised route extensions were never delivered. To truly enable optimal transportation efficiency, good urban development must be an ongoing priority with sustained investment.
We rely on a great number of material goods in our lives, and the freight industry plays a critical role in transporting these materials and goods to where they are needed. Yet at the same time, freight transportation makes up 8 percent of global greenhouse gas emissions, a majority of which comes from road vehicles such as trucks.
The way to improve trucking efficiency is by replacing inefficient trucks with more efficient ones, right? Certainly, the emergence of cost-competitive electric trucks will be a major help. But while more efficient trucks are a big part of the solution, focusing on trucks alone obscures many other opportunities for efficiency improvement in the freight transportation system, ranging from driver behavior to the overall shape of our future economy. Together, these other levers could account for a large share of the overall savings opportunity.
Material Demand Reduction
The starting point for reducing trucking emissions is not in the trucking sector, or even the larger freight sector, but rather in basic assumptions about our future economy. One possibility comes from the Low Energy Demand (LED) scenario developed by the International Institute for Applied Systems Analysis (IIASA), which provides a widely referenced benchmark for a low-energy-demand economy. LED assesses how the quantities and types of services drive supply and requirements for energy, materials, and land. Solutions include familiar measures like product lightweighting (e.g., smaller, lighter cars), fewer cars, and more efficient use of residential floorspace — as well as additional measures such as increased product longevity, sharing and service business models, reduced loss and waste, and increased material efficiency. All these measures reduce the quantity and volume of goods that must be moved, while maintaining or improving human well-being.
There are solutions across sectors, including the freight system itself. For example, Walmart’s whole-systems approach has included working with supply chain partners to lessen the volume of packaging, lighten certain cargo, improve truck loading techniques, and more.
Combined with modal shifts to rail, which we discuss below, LED shows that freight activity can be reduced to less than half of business-as-usual scenarios such as the International Energy Agency’s Stated Policies Scenario, or STEPS (Exhibit 5).
Freight Network Improvements
In parallel with material demand reduction, there are additional opportunities to reduce trucking demand through changes to the overall freight system.
Notably, shifting freight from truck to rail can lead to significant energy and cost savings, as trucks consume nearly three times more energy per ton-km as compared with rail. While this sounds straightforward, facilitating this modal shift is complex — often because today’s long-distance rail freight routes require high shipment densities to be profitable. Solutions include the integration of long-distance and short-line rail, aided by the build-out of smaller, low-cost intermodal hubs (for rail-to-truck transfer), or logistics hubs that consolidate freight volumes across multiple firms and industries. These hubs can also help improve route optimization and reduce the distance that trucks travel empty or below capacity.
Another solution is regional trucking, which can enable more direct routes and reduce miles traveled by as much as a third when combined with practices such as local warehousing and relay trucking — and can even reduce idling time.
Battery Electric Trucks and E-Cargo Bikes
By enabling shorter, simpler trucking routes, these network improvements go hand-in-hand with electrification. Electric trucks are currently better suited to shorter routes and require reliable charging availability, though this may change as battery technologies improve. Electric trucks elevate efficiency because they can be three to seven times more efficient than diesel trucks. Depending on the electricity source, this translates to a 60–80 percent reduction in emissions.
For last-mile deliveries, e-cargo bikes and electric three-wheelers provide significant energy savings, including in many emerging market economies. E-cargo bikes, for example, use 90 percent less energy per kilometer compared with an electric van, and they provide other benefits such as improved pedestrian safety and reduced congestion.
Driver Performance
Finally, where trucking cannot be reduced or avoided, we can improve efficiency by improving driver behavior. The fuel economy of trucks is greatly influenced by factors that drivers can control, such as speed, acceleration, braking, gear selection, idling, and more. Research shows that drivers can affect fuel efficiency by more than 20 percent. There are many ways to improve driver performance, including via training, incentives, and real-time driver feedback through telematic systems.
Exhibit 5. Impacts of End-Use Efficiency Measures on Global Energy Demand from Freight Trucking in 2050
Cultivating the Efficiency Mindset in the Trucking Industry The North American Council on Freight Efficiency (NACFE) is a fuel-agnostic organization focused on promoting the development and adoption of energy efficiency in the freight industry. NACFE shows how industry partners can help to increase awareness about efficiency-related cost savings, share and disseminate learnings about best practices, and create an industry-wide culture of continuous improvement.
Since 2017, in collaboration with RMI, NACFE has hosted four high-level demonstration events (NACFE Run on Less) aimed at showcasing the feasibility and impact of various trucking efficiency measures. The first two events highlighted the full savings potential from existing technologies, including for long-haul trucks and regional haul trucks. The 2021 and 2023 Run on Less events focused on battery electric trucks and truck charging depots, showcasing how fleets can maximize their benefits and scalability.
Each event has incorporated timely issues for the trucking industry, such as the growing regional haul market and the falling costs and weights of batteries. In addition, all demonstrations have involved a wide variety of trucks in terms of route, geography, vehicle type, and use case. In doing so, they have successfully called the trucking industry’s attention to effective, ready-for-market technologies, dispelling common misunderstandings and myths.
Beyond the Run on Less events, NACFE also issues confidence reports on established technologies and guidance reports on emerging technologies, as well as hosting regular trainings and workshops to help fleet owners and other relevant stakeholders make informed decisions.
Avoiding steel emissions by reducing our need for steel
Steel is one of the largest greenhouse gas emitters in heavy industry. Accordingly, new policies have been advanced in the United States, the European Union, India, and Australia to accelerate “green” steel production through direct subsidies, export credits and loans, green procurement, and penalties for emitting CO2 from traditional steelmaking. While green steel is an important way to reduce greenhouse gas emissions, there are other potent levers to reduce the emissions associated with iron and steel while respecting planetary boundaries. Starting with the end in mind, let’s consider how and why steel is used and assess a fuller range of efficiency solutions — including demand-side levers, materials efficiency, and circularity.
How does steel contribute to our well-being? The biggest uses of steel are in buildings, vehicle manufacturing, and transport infrastructure. So, how could our cities, homes, and mobility evolve to affect the demand for steel?
Reducing Steel Demand
How much steel do we need? Potentially only half of what we think.
One study concludes that global energy demand for steel production could be 60 percent lower than in a business-as-usual scenario in 2050 (Exhibit 6) when taking full advantage of demand-side approaches such as the following:
- Material efficiency focuses on providing the same material services with fewer materials. For example, smaller cars use less steel and other materials than SUVs or pickup trucks, but they can equally fulfill day-to-day needs in most cases.
- Material substitution aims to substitute away from energy-intensive materials such as steel and concrete. For example, steel can be replaced with bio-based structural materials or advanced synthetic materials.
- Material circularity reduces energy and resources by using recycled materials. For instance, producing a unit of recycled steel uses only 10–25 percent as much energy as producing virgin steel. This is primarily because virgin steel production requires an extra step of processing (iron ore reduction) that is not required when recycling scrap steel.
Separately, Mission Possible Partnership’s Steel Transition Strategy, endorsed by major global steel manufacturers, concluded that steel demand could be reduced by 41 percent in 2050 via circularity, productivity of use, and material efficiency alone.
Green Steel Production
Where primary steel production cannot be avoided, novel iron reduction and steelmaking methods can substantially reduce emissions. Best known today is hydrogen-based direct reduction, which uses green hydrogen produced from renewable energy, but there are other emerging technologies such as molten oxide electrolysis, electrowinning, and smelting via hydrogen plasma or lasers. These methods are still very energy-intensive — highlighting the importance of other efficiency measures alongside continued improvements of these methods themselves — but they can be fully powered by renewable electricity, so they offer the best path forward to reduce emissions from primary steel production.
Exhibit 6. Impacts of End-Use Efficiency Measures on Global Energy Demand from Steel Production in 2050
Industrial Clusters and Supply Chains
The development of industrial hubs and clusters offers additional system-level opportunities to reduce energy demand and emissions from steel. For example, industrial clusters could up-level energy efficiency by repurposing waste heat and materials, sharing infrastructure, and other synergies. The energy savings from such “industrial symbiosis” across steel, cement, aluminum, and paper production could be as high as 18 percent.
Industrial hubs will become critical as steel production shifts to green hydrogen. Many planned green steel factories will be sited near hydrogen hubs. For example, the Pilbara Hydrogen Hub in Australia will colocate green steel facilities with hydrogen production and export facilities. This can enable new modes of industrial symbiosis around green hydrogen, as well as create opportunities for cost and energy savings in transporting steel, as explained in the box below.
Reimagining the Steel Value ChainThe Green Iron Corridors project, led by RMI and the Green Hydrogen Catapult, envisions a radical restructuring of the steel value chain that will enable rapid growth of green steel while delivering significant cost and energy savings.
Traditionally, steel has been produced in large integrated facilities that include both the ironmaking and steelmaking process. This means that steel production is currently sited where coal and iron ore — both key inputs for traditional steelmaking — are cheap.
However, with the rise of green steel made using green hydrogen, the calculus changes. The Green Iron Corridors project proposes splitting up the ironmaking and steelmaking processes, with ironmaking sited in locations with abundant iron ore and renewable energy, such as Australia, and steelmaking in locations with existing manufacturing capabilities and strong demand markets, such as Germany.
Transforming this value chain not only facilitates the growth of green steel, but also improves energy efficiency by avoiding unnecessary transport. Rather than shipping both iron ore and hydrogen (via an ammonia carrier), importing a finished green iron product could save up to 3.1 TWh of total electricity use for a commercial-scale plant producing two million tons of steel per year (even considering the necessary reheating step to produce steel from cold iron). Producing green hydrogen in renewable-rich regions is also more infrastructure-efficient than doing so in traditional demand centers: completely replacing the EU’s nearly 100 million tons of traditional, integrated, carbon-intensive production with green production would require 70 GW less renewable capacity in Western Australia than it would in Germany.
Multiple benefits: Lower emissions, costs, and pollution
The energy efficiency opportunities described here align with a common pattern. In each case, significant savings can come from technology change — from green steel to electrification in buildings, cars, and freight. And in each example, energy end-use efficiency can bring further savings while facilitating the adoption of new technologies.
However, energy end-use efficiency is also powerful because it has cross-cutting benefits across sectors. Exhibit 7 summarizes many of the efficiency measures we’ve highlighted, emphasizing the interconnections in yellow. Urban land use and transportation policies and planning should go hand in hand, with efficiency implications for buildings, transportation, and even the steel sector and beyond — since fewer cars means less material demand for car-related infrastructure. Similarly, material efficiency, substitution, and circularity practices can reduce demand for steel while relieving freight burdens. Less freight, in turn, contributes to less traffic — further reducing demand for transportation infrastructure.
The benefits of these efficiency measures extend beyond energy savings to human well-being, as we discuss in a separate article. Fewer cars means less traffic, which means improved air quality and lower risk of traffic accidents. More walking and cycling mean better health outcomes. Passive housing design can increase household resilience to severe weather events.
In short, energy end-use efficiency measures are systemic leverage points where one intervention can lead to broader benefits for human well-being — which is why, after all, we use energy in the first place.
Exhibit 7. The Cross-Cutting Benefits of Energy End-Use Efficiency Measures

Delivering on global goals to double energy efficiency improvement rates will not only require major technology transitions in key sectors, but also better planning, design, operation, system integration, and circularity. As Amory Lovins writes, “saving energy is like eating a lobster: if you extract only the large chunks of meat from the tail and claws and throw away the rest, you will miss a comparable amount of tasty morsels tucked in crevices.”
These changes, in turn, depend on a diverse range of actors including policymakers, private companies, civil society, and consumers themselves, supported by a social norm that values efficiency and sufficiency. Establishing this kind of awareness provides an ethic of care that inspires and aligns actions at many different levels, including strengthening support for government policies and motivating corporate energy efficiency efforts.
Valuing EfficiencyThe idea of frugality may seem passé in the context of our supercharged, consumption-oriented global economy, but some leaders today are invoking this value as a key part of the path to greater well-being. Researchers working on energy and climate have chosen the more analytical term high-with-low to describe pathways to sustainability that center demand-side solutions for high well-being with low resource demand. These pathways achieve high levels of human development while keeping human resource footprints within planetary boundaries. Research shows that while demand-side solutions are generally underemphasized in the analysis of energy transition pathways, high-with-low scenarios show great promise for delivering a sustainable future while mitigating the worst consequences of climate change.
Is it possible to revive efficiency as a social norm? Values such as efficiency and frugality have deep and cross-cultural roots. Confucius promoted moderation as central to leading a virtuous life; he believed that practicing frugality was a way to cultivate moral character, humility, and responsibility. Mohandas Gandhi said, “The world has enough for everyone’s need, but not enough for everyone’s greed” and encouraged all people to “live simply so that others may simply live.” In the early history of the United States, Benjamin Franklin coined the phrase “a penny saved is a penny earned,” and he made the ethic of frugality a constant theme of his writings in Poor Richard’s Almanac, published to the 13 colonies for over 25 years from 1732 to 1758. “Without industry and frugality nothing will do; with them, everything,” Franklin wrote.
Given the diversity of actors involved in delivering energy efficiency, refreshing the societal values of efficiency and frugality can be an important force to reinforce these actions. Is a social tipping point in this direction possible?
Here are some examples of existing actions to highlight the energy efficiency resource — and the benefits of unlocking it:
- Global: Leaders of 197 nations plus the European Union signed a Framework Convention at COP28 setting a goal to double the rate of energy efficiency improvement between the time of the agreement and 2030. The agreement raised the profile of energy efficiency among national governments, energy and climate experts, and the media. Other global efforts have aligned with this goal, including Mission Efficiency — an alliance of partners from civil society and public- and private-sector institutions urging world leaders to unlock the energy efficiency investment that is crucial to meeting climate targets.
- National: Many countries have adopted national goals and/or launched campaigns to promote energy efficiency at a national or multinational level. These include India’s Lifestyles for Environment (LiFE), a mass movement championed by Prime Minister Modi to nudge individual and community action to protect and preserve the environment. “What is needed today is mindful and deliberate utilization, instead of mindless and destructive consumption,” says Modi. Today, over 1 million schools in India are connected to eco-clubs aimed at raising awareness about sustainable lifestyles. Other awareness campaigns include the EU’s NUDGE project, which implements and evaluates different behavioral interventions for energy efficiency, paving the way for new policies and increased consumer awareness, and Japan’s “Cool Biz” campaign that encourages weather-appropriate attire for office workers. Other countries such as New Zealand and Bhutan take a more holistic approach to encourage an energy efficient — or sufficient — lifestyle by encouraging a focus on well-being.
- City and community: Many cities and communities around the world have emphasized energy efficiency in their planning, policies, and outreach. One example of a community with an integrated approach to energy and resource efficiency is Palava City, which was mentioned above as an example of walkable urban design, with parallel efforts in energy, materials, and water efficiency. The city is designed to be a “living laboratory” for continuously refining and improving sustainability practices. Another example is the Vauban district of Freiburg, Germany, where every building has rooftop solar and many are built according to passive design principles. The city is built around walking, biking, and a tramway, and it has a sustainable waste management system.
- Corporate and industry: Businesses have established numerous coalitions to increase awareness of energy efficiency opportunities and accelerate implementation of solutions. For example, EP100 is a global corporate energy efficiency initiative, led by Climate Group, bringing together more than 125 ambitious businesses committed to improving their energy efficiency. EP100 members are committed to doubling their energy productivity, rolling out energy management systems, or achieving net-zero carbon buildings. By making public commitments, and driving toward those targets, companies can show climate leadership and send a powerful signal to policymakers and other businesses about the enormous potential of energy efficiency.
Acknowledgments
Special thanks to Ari Kahn, Kaitlyn Ramirez, Mike Roeth, Eva Rosenbloom, Lucas Toffoli, Brett Webster, Rachel Wilmoth, and Nick Yavorsky for their contributions.
The authors acknowledge support from the Energy Demand changes Induced by Technological and Social innovations (EDITS) project, which is part of the initiative coordinated by the Research Institute of Innovative Technology for the Earth (RITE) and the International Institute for Applied Systems Analysis (IIASA), funded by the Ministry of Economy, Trade, and Industry (METI), Japan.
Steel is one of the largest greenhouse gas emitters in heavy industry. Accordingly, new policies have been advanced in the United States, the European Union, India, and Australia to accelerate “green” steel production through direct subsidies, export credits and loans, green procurement, and penalties for emitting CO2 from traditional steelmaking. While green steel is an important way to reduce greenhouse gas emissions, there are other potent levers to reduce the emissions associated with iron and steel while respecting planetary boundaries. Starting with the end in mind, let’s consider how and why steel is used and assess a fuller range of efficiency solutions — including demand-side levers, materials efficiency, and circularity.
How does steel contribute to our well-being? The biggest uses of steel are in buildings, vehicle manufacturing, and transport infrastructure. So, how could our cities, homes, and mobility evolve to affect the demand for steel?
Reducing Steel Demand
How much steel do we need? Potentially only half of what we think.
One study concludes that global energy demand for steel production could be 60 percent lower than in a business-as-usual scenario in 2050 (Exhibit 6) when taking full advantage of demand-side approaches such as the following:
- Material efficiency focuses on providing the same material services with fewer materials. For example, smaller cars use less steel and other materials than SUVs or pickup trucks, but they can equally fulfill day-to-day needs in most cases.
- Material substitution aims to substitute away from energy-intensive materials such as steel and concrete. For example, steel can be replaced with bio-based structural materials or advanced synthetic materials.
- Material circularity reduces energy and resources by using recycled materials. For instance, producing a unit of recycled steel uses only 10–25 percent as much energy as producing virgin steel. This is primarily because virgin steel production requires an extra step of processing (iron ore reduction) that is not required when recycling scrap steel.
Separately, Mission Possible Partnership’s Steel Transition Strategy, endorsed by major global steel manufacturers, concluded that steel demand could be reduced by 41 percent in 2050 via circularity, productivity of use, and material efficiency alone.
Green Steel Production
Where primary steel production cannot be avoided, novel iron reduction and steelmaking methods can substantially reduce emissions. Best known today is hydrogen-based direct reduction, which uses green hydrogen produced from renewable energy, but there are other emerging technologies such as molten oxide electrolysis, electrowinning, and smelting via hydrogen plasma or lasers. These methods are still very energy-intensive — highlighting the importance of other efficiency measures alongside continued improvements of these methods themselves — but they can be fully powered by renewable electricity, so they offer the best path forward to reduce emissions from primary steel production.
Exhibit 6. Impacts of End-Use Efficiency Measures on Global Energy Demand from Steel Production in 2050
Industrial Clusters and Supply Chains
The development of industrial hubs and clusters offers additional system-level opportunities to reduce energy demand and emissions from steel. For example, industrial clusters could up-level energy efficiency by repurposing waste heat and materials, sharing infrastructure, and other synergies. The energy savings from such “industrial symbiosis” across steel, cement, aluminum, and paper production could be as high as 18 percent.
Industrial hubs will become critical as steel production shifts to green hydrogen. Many planned green steel factories will be sited near hydrogen hubs. For example, the Pilbara Hydrogen Hub in Australia will colocate green steel facilities with hydrogen production and export facilities. This can enable new modes of industrial symbiosis around green hydrogen, as well as create opportunities for cost and energy savings in transporting steel, as explained in the box below.
Reimagining the Steel Value ChainThe Green Iron Corridors project, led by RMI and the Green Hydrogen Catapult, envisions a radical restructuring of the steel value chain that will enable rapid growth of green steel while delivering significant cost and energy savings.
Traditionally, steel has been produced in large integrated facilities that include both the ironmaking and steelmaking process. This means that steel production is currently sited where coal and iron ore — both key inputs for traditional steelmaking — are cheap.
However, with the rise of green steel made using green hydrogen, the calculus changes. The Green Iron Corridors project proposes splitting up the ironmaking and steelmaking processes, with ironmaking sited in locations with abundant iron ore and renewable energy, such as Australia, and steelmaking in locations with existing manufacturing capabilities and strong demand markets, such as Germany.
Transforming this value chain not only facilitates the growth of green steel, but also improves energy efficiency by avoiding unnecessary transport. Rather than shipping both iron ore and hydrogen (via an ammonia carrier), importing a finished green iron product could save up to 3.1 TWh of total electricity use for a commercial-scale plant producing two million tons of steel per year (even considering the necessary reheating step to produce steel from cold iron). Producing green hydrogen in renewable-rich regions is also more infrastructure-efficient than doing so in traditional demand centers: completely replacing the EU’s nearly 100 million tons of traditional, integrated, carbon-intensive production with green production would require 70 GW less renewable capacity in Western Australia than it would in Germany.
The energy efficiency opportunities described here align with a common pattern. In each case, significant savings can come from technology change — from green steel to electrification in buildings, cars, and freight. And in each example, energy end-use efficiency can bring further savings while facilitating the adoption of new technologies.
However, energy end-use efficiency is also powerful because it has cross-cutting benefits across sectors. Exhibit 7 summarizes many of the efficiency measures we’ve highlighted, emphasizing the interconnections in yellow. Urban land use and transportation policies and planning should go hand in hand, with efficiency implications for buildings, transportation, and even the steel sector and beyond — since fewer cars means less material demand for car-related infrastructure. Similarly, material efficiency, substitution, and circularity practices can reduce demand for steel while relieving freight burdens. Less freight, in turn, contributes to less traffic — further reducing demand for transportation infrastructure.
The benefits of these efficiency measures extend beyond energy savings to human well-being, as we discuss in a separate article. Fewer cars means less traffic, which means improved air quality and lower risk of traffic accidents. More walking and cycling mean better health outcomes. Passive housing design can increase household resilience to severe weather events.
In short, energy end-use efficiency measures are systemic leverage points where one intervention can lead to broader benefits for human well-being — which is why, after all, we use energy in the first place.
Exhibit 7. The Cross-Cutting Benefits of Energy End-Use Efficiency Measures
Delivering on global goals to double energy efficiency improvement rates will not only require major technology transitions in key sectors, but also better planning, design, operation, system integration, and circularity. As Amory Lovins writes, “saving energy is like eating a lobster: if you extract only the large chunks of meat from the tail and claws and throw away the rest, you will miss a comparable amount of tasty morsels tucked in crevices.”
These changes, in turn, depend on a diverse range of actors including policymakers, private companies, civil society, and consumers themselves, supported by a social norm that values efficiency and sufficiency. Establishing this kind of awareness provides an ethic of care that inspires and aligns actions at many different levels, including strengthening support for government policies and motivating corporate energy efficiency efforts.
Valuing EfficiencyThe idea of frugality may seem passé in the context of our supercharged, consumption-oriented global economy, but some leaders today are invoking this value as a key part of the path to greater well-being. Researchers working on energy and climate have chosen the more analytical term high-with-low to describe pathways to sustainability that center demand-side solutions for high well-being with low resource demand. These pathways achieve high levels of human development while keeping human resource footprints within planetary boundaries. Research shows that while demand-side solutions are generally underemphasized in the analysis of energy transition pathways, high-with-low scenarios show great promise for delivering a sustainable future while mitigating the worst consequences of climate change.
Is it possible to revive efficiency as a social norm? Values such as efficiency and frugality have deep and cross-cultural roots. Confucius promoted moderation as central to leading a virtuous life; he believed that practicing frugality was a way to cultivate moral character, humility, and responsibility. Mohandas Gandhi said, “The world has enough for everyone’s need, but not enough for everyone’s greed” and encouraged all people to “live simply so that others may simply live.” In the early history of the United States, Benjamin Franklin coined the phrase “a penny saved is a penny earned,” and he made the ethic of frugality a constant theme of his writings in Poor Richard’s Almanac, published to the 13 colonies for over 25 years from 1732 to 1758. “Without industry and frugality nothing will do; with them, everything,” Franklin wrote.
Given the diversity of actors involved in delivering energy efficiency, refreshing the societal values of efficiency and frugality can be an important force to reinforce these actions. Is a social tipping point in this direction possible?
Here are some examples of existing actions to highlight the energy efficiency resource — and the benefits of unlocking it:
- Global: Leaders of 197 nations plus the European Union signed a Framework Convention at COP28 setting a goal to double the rate of energy efficiency improvement between the time of the agreement and 2030. The agreement raised the profile of energy efficiency among national governments, energy and climate experts, and the media. Other global efforts have aligned with this goal, including Mission Efficiency — an alliance of partners from civil society and public- and private-sector institutions urging world leaders to unlock the energy efficiency investment that is crucial to meeting climate targets.
- National: Many countries have adopted national goals and/or launched campaigns to promote energy efficiency at a national or multinational level. These include India’s Lifestyles for Environment (LiFE), a mass movement championed by Prime Minister Modi to nudge individual and community action to protect and preserve the environment. “What is needed today is mindful and deliberate utilization, instead of mindless and destructive consumption,” says Modi. Today, over 1 million schools in India are connected to eco-clubs aimed at raising awareness about sustainable lifestyles. Other awareness campaigns include the EU’s NUDGE project, which implements and evaluates different behavioral interventions for energy efficiency, paving the way for new policies and increased consumer awareness, and Japan’s “Cool Biz” campaign that encourages weather-appropriate attire for office workers. Other countries such as New Zealand and Bhutan take a more holistic approach to encourage an energy efficient — or sufficient — lifestyle by encouraging a focus on well-being.
- City and community: Many cities and communities around the world have emphasized energy efficiency in their planning, policies, and outreach. One example of a community with an integrated approach to energy and resource efficiency is Palava City, which was mentioned above as an example of walkable urban design, with parallel efforts in energy, materials, and water efficiency. The city is designed to be a “living laboratory” for continuously refining and improving sustainability practices. Another example is the Vauban district of Freiburg, Germany, where every building has rooftop solar and many are built according to passive design principles. The city is built around walking, biking, and a tramway, and it has a sustainable waste management system.
- Corporate and industry: Businesses have established numerous coalitions to increase awareness of energy efficiency opportunities and accelerate implementation of solutions. For example, EP100 is a global corporate energy efficiency initiative, led by Climate Group, bringing together more than 125 ambitious businesses committed to improving their energy efficiency. EP100 members are committed to doubling their energy productivity, rolling out energy management systems, or achieving net-zero carbon buildings. By making public commitments, and driving toward those targets, companies can show climate leadership and send a powerful signal to policymakers and other businesses about the enormous potential of energy efficiency.
Acknowledgments
Special thanks to Ari Kahn, Kaitlyn Ramirez, Mike Roeth, Eva Rosenbloom, Lucas Toffoli, Brett Webster, Rachel Wilmoth, and Nick Yavorsky for their contributions.
The authors acknowledge support from the Energy Demand changes Induced by Technological and Social innovations (EDITS) project, which is part of the initiative coordinated by the Research Institute of Innovative Technology for the Earth (RITE) and the International Institute for Applied Systems Analysis (IIASA), funded by the Ministry of Economy, Trade, and Industry (METI), Japan.
Special thanks to Ari Kahn, Kaitlyn Ramirez, Mike Roeth, Eva Rosenbloom, Lucas Toffoli, Brett Webster, Rachel Wilmoth, and Nick Yavorsky for their contributions.
The authors acknowledge support from the Energy Demand changes Induced by Technological and Social innovations (EDITS) project, which is part of the initiative coordinated by the Research Institute of Innovative Technology for the Earth (RITE) and the International Institute for Applied Systems Analysis (IIASA), funded by the Ministry of Economy, Trade, and Industry (METI), Japan.